Tired Worms Suggest Sleep Is an Emergent Property of Neural Networks
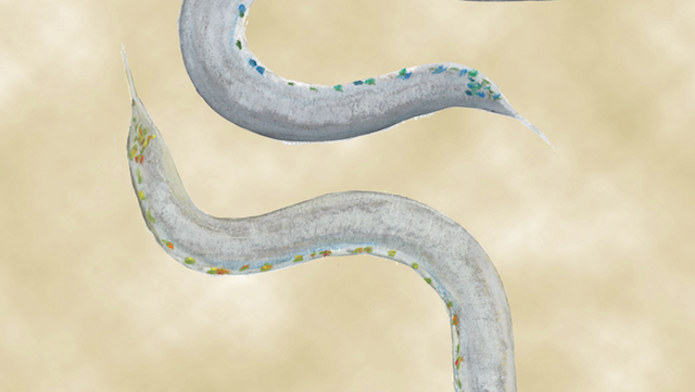
Sleep is ubiquitous across the animal kingdom — all species assessed to date show some kind of sleep state. But no one knows the precise purpose of sleep. “Why does every neural network need some relaxation?” asks Manuel Zimmer, a neuroscientist at the Research Institute of Molecular Pathology, in Vienna, and an investigator with the Simons Collaboration on the Global Brain. “Neuroscience has a lot of good hypotheses but not a unifying clear-cut answer to why that’s the case.”
Zimmer and collaborators aim to chip away at this question using newly developed techniques to monitor the whole brain in the microscopic round worm C. elegans as it falls asleep and wakes up. “That makes it possible to watch how the brain switches between these drastically different states,” Zimmer says.
In a paper published in Science in June, Zimmer and collaborators characterize a neurological sleep state in worms. The study is the first study to do so in invertebrates — previous work had defined invertebrate sleep via behavior, such as lack of movement. The findings show that the brain is largely quiescent during sleep. Analysis of neural dynamics as animals fall asleep support the idea that sleep is an emergent property of neural networks. “I think this is some of the stronger evidence for it,” Zimmer says.
Neuroscientists have different ways to conceive of sleep. Classic studies from the early 20th century showed that depriving the brain of sensory input induced a sleep-like state, suggesting that sleep represents the brain’s resting state. “But the discovery of REM sleep in 1950s, where the brain is extremely active during sleep, led to a change in thinking,” says David Raizen, a neuroscientist at the University of Pennsylvania. Scientists theorized that the sleeping nervous system is still active, just in a different physiological state than when awake.
In recent years, however, new evidence has emerged for sleep as a default resting state — neurons grown in culture spontaneously enter a sleep-like state of electrical activity. “They fire in a slow synchronized fashion, which is what the brain does in sleep, suggesting that sleep is a ground physiological state of neurons,” Raizen says. Zimmer’s new findings support this idea as well, the first such support from a live animal. “This paper reintroduces the concept that sleep is a ground state that the brain enters in the absence of stimulation,” Raizen says.
A worm lullaby
Worms tend to sleep most during a certain stage of development rather than according to a circadian cycle like humans or mice. Zimmer and his coworkers have shown that low oxygen, around 10 percent, is sufficient to allow worms in the appropriate developmental state to sleep, whereas standard atmospheric levels, about 21 percent, wakes them up. In the new study, Zimmer’s team used varying oxygen levels to cycle the animals in and out of sleep. “It’s a powerful experimental paradigm,” Zimmer says. “Combined with our whole brain imaging technique, we can watch the entire brain as the worm falls asleep and wakes up.” Zimmer notes that a 10 percent oxygen level isn’t noxious to worms, which live in the soil and are adapted to low oxygen environments.
By clicking to watch this video, you agree to our privacy policy.
Under normal waking conditions, C. elegans’ neurons are quite active. The new research shows that when the animal falls asleep, roughly three quarters of normally active neurons go silent. “We were surprised by how drastic it was,” Zimmer says.
A handful of neurons remain active during sleep, however. These include neurons that secrete the neurotransmitter GABA, which has been linked to sleep in mammals. One particular neuron, known as RIS, is active when worms are awake and becomes more active as the animals grow sleepy. Researchers found that when RIS activity rises above a certain threshold, the worm is more likely to fall asleep. “It seems to be an indicator of how tired the brain is,” Zimmer says.
Biased toward sleep
Zimmer and collaborators initially theorized that high RIS neuron activity would drive the network into a sleep state. But analysis of neural dynamics suggests a subtler transition. RIS activity seems to nudge the network into a state of quiescence, biasing it toward sleep.
In the language of complex dynamical systems, sleep act likes an attractor state; if left unperturbed — in this case, without the sensory stimulation of extra oxygen — the worm’s brain naturally settles into a sleep state. Zimmer likens this state to a valley in a hilly landscape. A marble rolling through the landscape will likely fall into the hole. When the worm is in an environment and developmental stage conducive to sleep, it needs constant arousal — the equivalent of someone pushing the marble up a hill — to remain awake. When the worm is no longer tired, the sleep valley is much shallower, making the marble less likely to get trapped.
Zimmer says it makes sense for sleep to unfold through this type of mechanism. If sleep neurons gently modify the network to create a sleepy state, it’s easy to switch the network back to wakefulness. “It’s an efficient way to switch the brain between sleep and wakefulness,” he says. “Subtle modulation of the network can have a drastic effect, which means you need less energy to get the system awake again.”
A system in which the RIS neuron had a more direct role in inducing sleep, inhibiting other neurons in the network for example, would be more difficult to revert to wakefulness. In the event of a predator or some other danger, “the network would need to overcome the sleep-induced inhibition or wash away some sleep factor in order to awaken,” Zimmer says.
The purpose of sleep
Worms, which have a few hundred neurons, are clearly very different from mammals. But Zimmer thinks the fundamental principle — the attractor mechanism — at work in worm sleep can operate in larger neural networks as well.
Moreover, the apparent deep evolutionary roots of sleep suggest that lessons in one species will apply to another. “Based on the idea that sleep is phylogenetically ancient, I would argue that the function of sleep — what drove its evolution despite the costs — would occur in both worms and mammals,” Raizen says.
Zimmer’s team next aims to decipher the attractor mechanism underlying sleep. They will use optogenetics to control activity of specific neurons and measure how this affects sleep.
They also plan to examine some of the broader questions around sleep. “We have a window into one of the most fascinating questions in neuroscience” Zimmer says. “We can deprive worms of sleep and watch what goes wrong in the brain at the level of single neurons. And we can let the worm go back to sleep and see how that gets repaired.”