Heavy-Metal Mysteries in the Midst of Exploding Stars
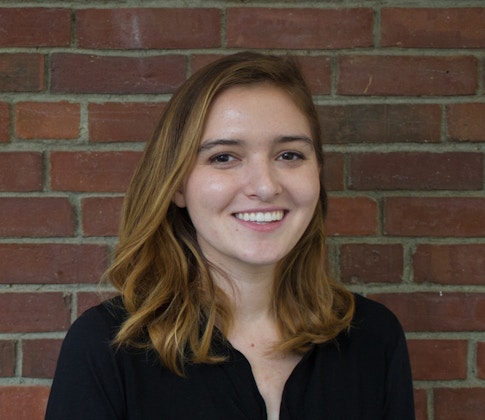
For many people, stargazing offers the chance to pick out constellations or contemplate their place in the universe. For astrophysicist Ashley Villar, looking at stars takes on a tone that is perhaps psychologically darker but physically brighter: “I study the eruptions, collisions and explosions of stars — all the ways that stars can die,” she says.
Villar, a member of the 2020 class of the Simons Society of Fellows, finds stellar deaths fascinating because they forge the heaviest metals in the universe in ways that are poorly understood. Villar develops machine-learning tools to make sense of a torrent of astronomical data collected from around the world that has grown significantly in recent years, thanks to new data-gathering techniques. The amount will grow still more when a new observatory opens in Chile in a few years.
Villar, who has a Ph.D. from Harvard University, began one year of postdoctoral research at Columbia University this July. After that she is off to teach the next generation of astrophysicists, at Penn State University. I caught up with her just before her postdoc began to ask about her research. An edited version of our conversation follows.
Why are stellar explosions important?
Elements that are heavier than hydrogen, such as iron or gold, live in the bellies of stars. And the only way those elements enter and enrich our universe is when stars explode. I study exploding stars, which are known as supernovae.
What is the chemistry behind the creation of heavy elements in supernovae?
Heavy elements form through a chemical process known as the rapid neutron capture process, or the ‘r-process.’ But sometimes the heaviest elements, such as plutonium, do not form during this process; it seems that matters get ‘stuck’ at making elements that are not as heavy, like gold. By seeing one explosion, we can’t really resolve the mystery of how the heaviest elements form. That’s why we need to see more of them.
What inspired your interest in astronomy?
When I was in eighth grade, I read Neil deGrasse Tyson’s Death by Black Hole, after seeing him on The Daily Show with Jon Stewart, and from then on, I knew I wanted to study astrophysics. When I was graduating high school, I sent Tyson a fan letter to thank him for inspiring this interest. He actually called me up to wish me well.
That’s a cool story. And what do we know now that we did not know before, thanks to studying supernovae?
Here is an example from 2017, which is pretty recent in my field. Before then we didn’t really know where the heaviest elements in the universe came from — elements that are as heavy as gold and platinum. At the time astronomers theorized that these heavy elements develop when stars known as neutron stars collide, but that collision had never been seen in real time before. In 2017 we actually saw neutron stars collide! That was thanks to the Laser Interferometer Gravitational-Wave Observatory, or LIGO, which picked up the subtle gravitational distortions set off by the collision.
During that experiment, LIGO provided a treasure map for astronomers around the world, which includes where in the sky to focus telescopes and how far away they are. Maps like these are based on the likelihood of observing different types of collisions: black hole colliding with black hole, neutron star colliding with neutron star, etc. The LIGO team prepared our map by comparing live gravitational wave signals to simulations of what was likely to happen during various collisions. They suggested we point our telescopes at areas around 130 million light-years away — which is actually pretty close, on a cosmic scale — because it was extremely likely that we would see two neutron stars collide. The gravitational wave signal lined up perfectly with theoretical models, a very surprising result that proved we had in fact witnessed a neutron star collision.
How are astronomers building on LIGO’s spectacular observation?
We will observe the effects of this collision for at least another decade, as it sends different kinds of waves into the galaxy. Infrared waves came first, followed soon after by radio waves that are visible for months to years.
Studying the effects of this one collision is just part of the story. We also want to know: Why do collisions occur at some times but not others? Why do these collisions happen in some types of galaxies more than others? We’ll always be answering questions like this.
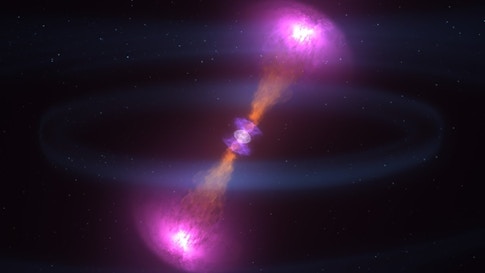
How do you study the data that comes from answering such questions?
Astronomers have a lot of big data to manage — a lot of it! But it’s not just about big data; what we call ‘wide data’ is another challenge: data that comes from all over the world, in all different formats, that then has to be organized so that it can be used. This is what I’ve been working on.
We discover 10,000 supernovae every year, but there’s not enough human hours to study them all. So I’m interested in developing machine-learning tools and statistical models that can help us study more supernovae than we are able to study now. This is important, because in just a few years, we’ll be able to detect up to two million supernovae a year when a new world-class observatory opens in Chile. That’s known as the Vera Rubin Observatory, and it is a collaboration between the United States and Chile. It was set to open in 2022, but that might be 2023 now because of the COVID-19 pandemic. When we’re able to spot that many supernovae, we’ll need algorithms that help us determine what is worth exploring in depth as well as statistical tools to make use of the data from explosions that we do not examine deeply.
How do you know which supernovae are worth exploring in depth, and which aren’t?
That’s a very philosophical question. To me, what’s most interesting would be something we truly have never seen before. So for every one million supernovae that we may see, what is the thing that only occurred literally one in a million times? It’s not crazy to think that there is still some astrophysics or even basic physics that we have yet to discover. Extreme anomalies could help us learn new things. This requires knowing what’s happened beforehand and identifying the new type of event in real time.
How has the work of astronomers changed during your time in graduate school?
When I began grad school, I would literally get on a plane, fly to Chile for 24 hours, and stay up all night and observe. Today we have a more automated process. A telescope is always scanning across the night sky, and there’s an automatic code that looks through the data for something new. Once that code flags something as potentially new, machine-learning tools that we’ve built apply another filter to see what’s really new. Then I get an email that says, “Hey, here’s something that might be interesting.” And then we figure out what’s happening.
What does this new era of big data mean to you?
I think about this new era of big data as like needles in a haystack. Machine learning can help find the needles — that once-in-a-million event that it would be hard to notice with just a telescope — and also help make sense of the ‘haystack.’ Countless other more typical cosmic events need to be labeled and classified, in terms of what types of supernovae they are. Right now we can label thousands of these events at a time; soon it will be millions, thanks to the Rubin Observatory. That’s enough data to answer new questions about supernovae: What types of stars do they come from? What did they do in the few years before they died? Did one star live with a ‘friend’ or another astronomical body that was within its orbit? It used to be hard to answer these questions. Now it will be easier.