Mathematics Explains How Giant Whirlpools Form in Developing Egg Cells
By clicking to watch this video, you agree to our privacy policy.
Egg cells are among the largest cells in the animal kingdom. If moved only by the random jostlings of water molecules, a protein could take hours or even days to drift from one side of a forming egg cell to the other. Luckily, nature has developed a faster way: cell-spanning whirlpools in the immature egg cells of animals such as mice, zebrafish and fruit flies. These vortices enable cross-cell commutes that take just a fraction of the time. But until now, scientists didn’t know how these crucial flows formed.
Using mathematical modeling, researchers now have an answer. The gyres result from the collective behavior of rodlike molecular tubes called microtubules that extend inward from the cells’ membranes, the researchers report on January 13 in Physical Review Letters.
“While much is not understood about the biological function of these flows, they distribute nutrients and other factors that organize the body plan and guide development,” says study co-lead author David Stein, a research scientist at the Flatiron Institute’s Center for Computational Biology (CCB) in New York City. Given how widely the swirling flows have been observed throughout the animal kingdom, “they are probably even in humans.”
Gabriele De Canio, a researcher at the University of Cambridge, co-led the study with Stein. Their co-authors were CCB director and New York University professor Michael Shelley and Cambridge professors Eric Lauga and Raymond Goldstein.
Scientists have studied cellular flows since the late 18th century, when the Italian physicist Bonaventura Corti peered inside cells using his microscope. He saw fluids in constant motion, but scientists didn’t understand the mechanisms driving these flows until the 20th century, when they identified the source of the motion: molecular motors that walk along the microtubules. Those motors haul large biological payloads such as lipids. Schlepping the cargo through a cell’s relatively thick fluids is like dragging a beach ball through honey. As the payloads move through the fluid, the fluid moves too, creating a small current.
But sometimes those currents aren’t so small. In certain developmental stages of a common fruit fly’s egg cell, scientists spotted whirlpool-like currents that spanned the entire cell. In these cells, microtubules extend inward from the cell’s membrane like stalks of wheat. Molecular motors climbing these microtubules push downward on the microtubule as they ascend. That downward force bends the microtubule, redirecting the resulting flows.
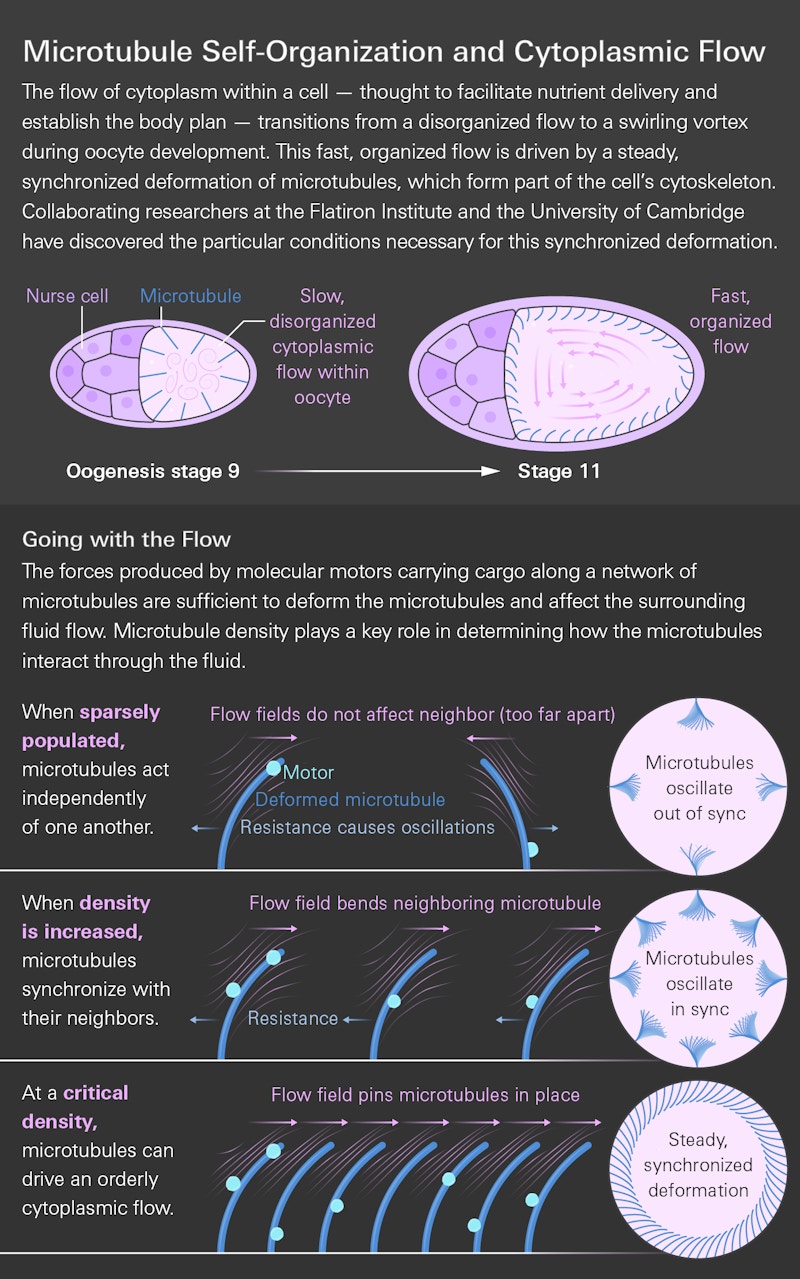
Previous studies considered this bending mechanism but applied it to isolated microtubules. Those studies predicted that the microtubules would wave around in circles, but such behavior didn’t match observations.
In the new study, the researchers added a key factor to their model: the influence of neighboring microtubules. That addition showed that the fluid flows generated by the payload-ferrying motors bend nearby microtubules in the same direction. With enough motors and a sufficient density of microtubules, all the microtubules eventually lean together like a wheat field caught in a strong breeze. This collective alignment orients all the flows in the same direction, creating the cell-wide vortex seen in real fruit fly cells.
While grounded in reality, the new model is stripped down to the bare essentials to reveal the conditions responsible for the swirling flows. The researchers are now working on versions that more realistically capture the physics behind the flows to better understand the role the currents play in biological processes.
Information for Press
For more information, please contact Stacey Greenebaum at [email protected].