Foundation Announces the Simons Collaboration on Extreme Electrodynamics of Compact Sources
The Simons Foundation is pleased to announce the establishment of the Simons Collaboration on Extreme Electrodynamics of Compact Sources (SCEECS) directed by Roger Blandford of Stanford University.
Recent, remarkable, astrophysical discoveries and upcoming experimental opportunities are confronting electrodynamics with new challenges that are taking it into new regimes, far beyond those where it can be used with confidence. Most of the astrophysical developments involve neutron stars and black holes. Collectively, they exhibit extreme electrodynamical conditions measured by magnetic field strengths beyond 100 GT (thirty times the QED Schwinger field), EMF above 100 ZV (ten billion times that associated with the Large Hadron Collider) and electromagnetic radiation with effective temperature as high as 1040K (comparable with that associated with a giant ocean wave). Photons and neutrinos are directly detected with energies in the PeV range and cosmic rays with energies up to ~0.2 ZeV. Our collaboration seeks to account for these observations by expanding the application of classical and quantum electrodynamics into these regimes.
Our neutron star program is centered upon magnetars which have the strongest magnetic field, captured by stars with radii about 10 km, made of nuclear matter with density of order a million billion times that of water. These have long been identified as sources of X-ray and g-ray flare. More recently, they have been with fast radio bursts leading us, inexorably, into new electrodynamical territory. We are addressing this challenge following a methodology that is both “bottom up” and “top down” to span a huge range of length scales. The first approach starts with quantum electrodynamics extended into the strong field and relativistic regime and uses kinetic simulations to capture collective plasma effects and the escape of photons. The second approach starts from the observations and uses large scale modeling, involving continuum mechanics, to interface with the microphysical view. In this way, it is hoped to choose between several competing models and to interface with a parallel investigation involving even stronger magnetic field inside the neutron star.
A similar procedure is being followed to explore the properties of stellar-size and galactic nuclear-size, spinning black holes, where electromagnetic field is now acknowledged as playing a major role in the dynamics of the disks of gas that feed them and the jets that they create. Here the electrodynamic frontiers involve the interface with general relativity and the acceleration of the highest energy cosmic rays. Recent observations by the Event Horizon Telescope and other observatories are revealing a rich variety of physical regimes. Our research on black holes is highly synergistic with that on neutron stars as both investigations include many similar fundamental processes and are carried out using similar types of simulation.
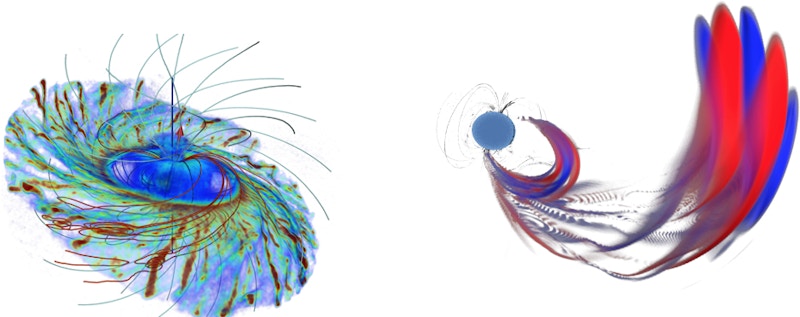
Left: “Particle in cell” simulation of the magnetic field and electric current associated with a spinning and strongly magnetized neutron star (adapted from Philippov and Kramer 2023). Right: Simulation of a powerful electromagnetic disturbance propagating out from the surface of neutron star following a magnetar quake and leading to a fast radio burst (adapted from Yuan et al. 2022).

Left: Event Horizon Telescope image of the massive black hole in the nucleus of the nearby galaxy M87 (adapted from EHT Collaboration 2022). The pattern of the inferred orbiting magnetic field lines is displayed. Right: Simulation of the magnetic field threading the black hole and confined by orbiting gas (adapted from Ripperda et al. 2022).