Singing Mice Hint at How Sensory Input Turns Into Behavior
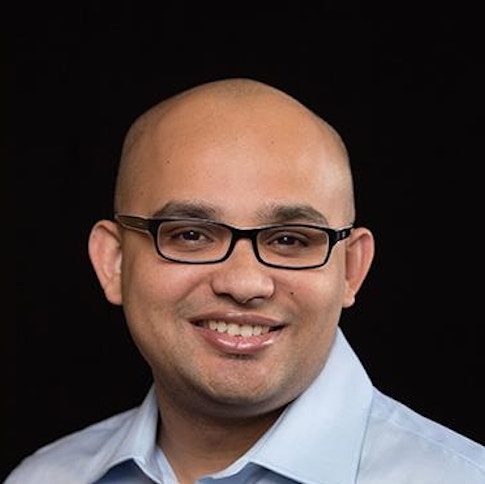
Arkarup Banerjee refers to himself as a biologist, but his interest in animal behavior didn’t start in the typical way. “I did not roam around in forests as a child, fascinated by snails — I never did any of that,” says Banerjee, who grew up in eastern India, in a suburb of Kolkata. Although “very comfortable” with physics and mathematics, he says he was drawn to biology and its inherent, emergent complexity: “how any behavior that you can possibly imagine is ultimately a result of molecules diffusing inside cells and communicating between themselves in a precise manner.” This bottom-up interest in organic systems led Banerjee to join the Tata Institute of Fundamental Research in Mumbai, where he helped build microscopes that could see molecules of dopamine passing between living neurons.
Now a Simons Society fellow in the Neuroscience Institute at the New York University Langone Medical Center, Banerjee investigates the neural circuits that enable pairs of Scotinomys teguina — also known as “Alston’s singing mice” — to vocalize to each other in a conversation-like way. An edited version of the interview follows.
Let’s start with the basics. What exactly are singing mice?
They’re quite different from laboratory mice, although they look like laboratory mice. They come from Central American rain forests. An important difference between these mice and Mus musculus is that these mice are diurnal and insectivorous. They are more active during the day and mostly eat insects in the wild. But in the lab, we feed them cat food.
We know that male mice will sing in the presence of females or when exposed to female odors. But more interestingly, they will often sing in response to another male’s song — a phenomenon known as counter-singing. They will actually wait for the other mouse to stop singing before responding with their own song in less than a second after the other stops. This is an innate behavior, and we don’t have to train them to do this.
What are you trying to learn about the brain from singing mice?
My motivation coming into Michael Long’s lab was to understand “sensorimotor transformations.” What do I mean by that? Think about what we are doing now: I am saying something, your auditory system has to interpret whatever I’m saying in real time, and then you have to respond appropriately. That’s the transformation: Your sensory stream is giving you constant input, and then somehow, the brain’s motor areas are able to act on that input, enabling you to react appropriately.
It turns out these singing mice are really, really great at this. Each mouse has to constantly keep track of the sensory information coming in and then respond, within a fraction of a second similar to human conversations.
More broadly, if you think about it, most behavior fundamentally can be boiled down to this idea of sensorimotor transformation. So figuring out how the brain is able to do this is a fundamental problem.
By clicking to watch this video, you agree to our privacy policy.
If sensorimotor transformation is so fundamental to animal behavior, why not use ordinary laboratory mice to study it?
Yes, it is indeed common to use laboratory mice to study this topic. Typically, what people do to investigate this problem is train mice to do a task: for example, if you hear a low-frequency tone, put your tongue towards the left; if you hear a high-frequency tone, put your tongue towards the right. By definition, this is a sensorimotor transformation, and by studying these tasks we have learned a lot about different brain circuits that are involved in such behaviors. The trouble is, mice never do this in the wild. Their brain circuits did not evolve to do this particular task. So, if you are interested in understanding those brain circuits that enable natural behaviors, you look for organisms that actually do sensorimotor transformations without having to train them.
What have you learned so far in your work?
To understand the brain, one needs to measure the brain’s output properly, which is behavior. So, I started with behavioral experiments to characterize the counter-singing behavior extensively — how often do these mice sing back, how quickly, et cetera. Daniel Okobi , a previous graduate student in the lab, had figured out that a particular part of the mouse’s motor cortex, when electrically stimulated or cooled, was able to influence the singing behavior. To test the role of that area, I did an experiment where I pharmacologically inactivated that region while the animals had to do their sensorimotor behavior of singing back and forth to each other.
We found that a mouse with this brain region temporarily inactivated was still able to sing his song just fine, but only “solo.” He could not couple his singing precisely to the sensory input of another mouse.
So that brain region appears to be involved in creating the sensorimotor transformation?
Exactly. The brain is a big place, so when you go looking for these mechanisms, the first level of the problem is: “Where do we start?” So we have figured out a good starting point.
You began your research career building microscopes. But in the 21st century, why do we still need new microscopes? Isn’t this a solved problem?
That’s a great question. If it’s just about being able to look at something small, we already have tools that can do it off the shelf. But being able to build your own microscopes to tailor the measurement in exactly the way you need it is very satisfying. For example, when I was a master’s degree student in Mumbai, I was interested in being able to catch these neurotransmitter molecules in action, inside real neurons, without artificially putting in an antibody or some kind of dye to label them. And no such technique existed. All the parts were available — lenses, mirrors, lasers. But how do you put them together? You come up with a new design that will allow you to do that. Moreover, it is much cheaper to build your own microscopes, and it is a lot of fun.
Is there an open question in your domain that you hope will be answered during the course of your lifetime?
It’s obvious that a grand question of this century is “How does the brain work?” Or to put it even more broadly, “What’s the nature of intelligence?” There are, of course, many intelligent problems that animals and humans solve, and now in parallel, artificial intelligence is able to solve problems that, 20 years ago, we would have considered exclusively the domains of extremely intelligent animals like humans.
I think a very interesting question is “What are the commonalities and differences between various biologically intelligent systems as well as artificially intelligent systems?” If you have only one example of how something works, then you really don’t know what the idiosyncrasies of that particular implementation are, versus the common principles. I think we are at this juncture where we have suddenly encountered artificial systems — computers — that can do equally well, if not better, on certain things. I want to understand, by the time I die perhaps, what the truly common principles are between intelligent systems of various kinds.