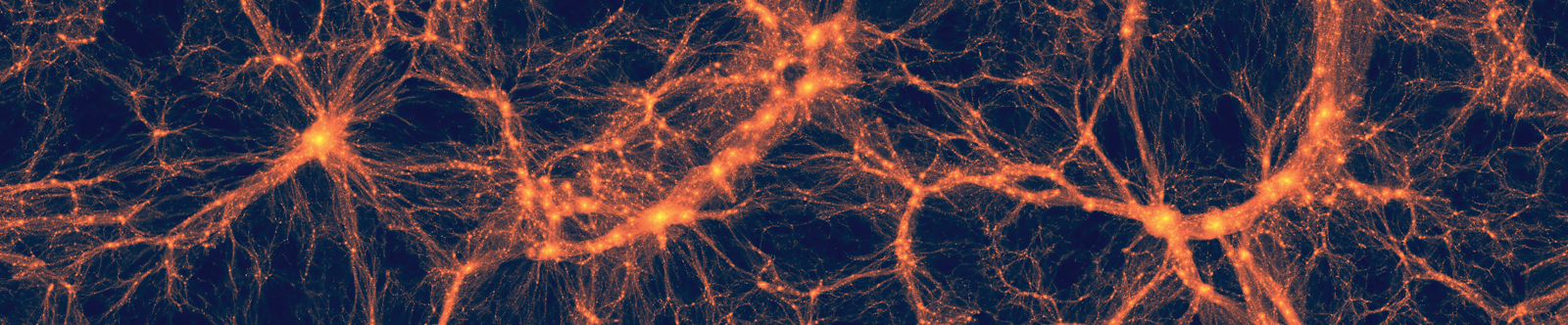
The Universe’s Dark Expanding Mystery
Is Earth similar in composition to the rest of the universe? For physicists and astronomers at the start of the 1900s, this seemed like a reasonable assumption. The Earth is composed primarily of carbon, silicon and iron; its oceans of hydrogen and oxygen; and its atmosphere of nitrogen and oxygen. Meteorites and comets have similar compositions. Over the past century, however, we have learned that this picture is rather incomplete. These elements make up less than 0.1 percent of the composition of the universe. Instead, 95 percent of the energy density of the universe is either in the form of dark matter (some new type of particle that has not yet been seen in any of our experiments) or dark energy (energy associated with empty space).
American astrophysicist Cecilia Payne led the first revolution in our understanding of the composition of the universe. In the 1920s, she applied the new physics of quantum mechanics to the spectra of the sun. In her controversial thesis, she showed that the sun is composed primarily of hydrogen and helium. Today, our measurements show that elements heavier than helium make up only 2 percent of the universe’s mass of ‘ordinary matter.’ The sun’s composition is very different from that of Earth: Iron, oxygen, silicon and magnesium make up more than 90 percent of Earth’s mass.
The second revolution in our understanding of the universe began with Swiss astronomer Fritz Zwicky’s observation of very rapid motions in clusters. Zwicky realized that ‘dunkle Materie’ (dark matter) was needed to bind the galaxies into clusters. In the 1970s, American astronomer Vera Rubin and her collaborators found that this dark matter was ubiquitous and dominated the outer regions of most galaxies. Over the past 20 years, cosmological observations, particularly measurements of the microwave background, have found five times more dark matter in the universe than ordinary matter.
What is this dark matter that dominates galaxies? Astronomers have searched for many plausible candidates: hot gas, cold gas, very low-mass stars, planets, even black holes. They have eliminated all of these ‘normal’ possibilities. Moreover, their observations have constrained the properties of the dark matter: It doesn’t interact strongly with protons or neutrons, it doesn’t have an electric charge, and it doesn’t interact much with neutrinos.
Cosmologists eventually realized that galaxies could not form unless the dark matter was ‘nonbaryonic’ — made of something new. Sherlock Holmes stated that, when you have eliminated the impossible, whatever remains, however improbable, must be the truth. The truth seems to be that most of the matter is made not of atoms or neutrinos, but of something else.
What is this ‘something else’? We still don’t know. Particle physicists have proposed several different possibilities: One is the lightest supersymmetric particle, which could be stable. It would have been created in the early universe and could be detected in deep underground experiments.
Another plausible dark matter candidate is axions. CASPEr, the Cosmic Axion Spin Precession Experiment, a Simons Foundation–supported project, is searching for these ultralight particles. CASPEr is sensitive to particles with masses 10-20th that of the electron. Axionic dark matter can cause nuclear spins to precess at a frequency set by the mass of the dark matter. If CASPEr sees these elusive axions, it will provide insights into not only the composition of the universe but also into physics at very high energy scales.
The Simons Observatory measurements of fluctuations in the cosmic microwave background will significantly improve our determination of the abundance of dark matter and also measure neutrinos to determine the energy density of the universe. In the early universe, the dark matter may have decayed into ordinary matter or been annihilated in such a way that it left behind ordinary matter; if so, the Simons Observatory will be sensitive enough to identify a signature of either decay or annihilation.

Over the past two decades, astronomers have discovered the observational signature of dark energy, an even more mysterious component of the universe. Dark energy is driving the expansion of the universe to accelerate ever faster. We see the signature of the effects of dark energy in the large-scale distribution of galaxies, through observations of distant supernovas and through observations of the microwave background. Should this acceleration continue, our universe will end as a very cold, empty place.
What is this dark energy? The most popular speculation is that dark energy is the energy associated with empty space, the so-called vacuum energy. If this explanation is correct, it means that physics at the tiniest quantum mechanical scales is driving the expansion of the whole universe. Astronomers are working to measure the effects of this dark energy on the expansion rate of the universe and on the growth of large-scale structures such as clusters of galaxies. By building the Large Synoptic Survey Telescope (LSST) and the Wide Field Infrared Survey Telescope (WFIRST), these scientists are working to survey the night sky in both the optical and the infrared. Their observations could reveal the nature of the dark energy; perhaps they could discover the breakdown of general relativity on the scale of the visual universe.
So far, the journey to discover the composition of the universe has mostly revealed our ignorance about its composition. Today, our best measurements imply that the elements that dominate Earth make up only 0.1 percent of the density of the universe. Hydrogen and helium account for the next 4.7 percent. The dark matter makes up 24 percent of the universe. The rest is dark energy. The challenge now is to determine the nature of 95 percent of the universe — to shed light on the part that remains dark.