
Studying Human Speech With Animals That Can’t Talk

Studying speech, that most human of attributes, is challenging for neuroscientists. Access to the human brain is largely limited to noninvasive brain imaging, which provides a coarse view of the areas involved in language. On rare occasions, scientists get brief, direct glimpses into the brain during surgery — surgeons use electrical stimulation to find brain areas involved in speech and other essential functions to avoid damaging those areas during the procedure. Researchers can incorporate experiments into these surgical studies, providing a valuable view of the inner workings of language and other cognitive functions.
Michael Long, a neuroscientist at the New York University School of Medicine, combines these rare human experiments with research on animal models to more deeply explore the neural circuitry of vocal communication. He focuses on two essential components of speech — vocal imitation and vocal interactions, or ‘turn-taking’ in conversations.
Songbirds, which learn complex songs from their fathers, provide a model of vocal learning. Long and collaborators have used techniques developed in the zebra finch to help map language circuits in the human brain. Another helpful species is new to neuroscience labs: a rodent from Costa Rica known as Alston’s singing mouse. These mice sing to each other in a rapid back-and-forth resembling human conversation, granting scientists an opportunity to explore the social aspect of speech. This research has inspired new experiments in human speech, shedding light on Broca’s area, a poorly understood brain region that was first tied to language more than 150 years ago.
Long, an investigator with the Simons Collaboration on the Global Brain, described his work at the Society for Neuroscience meeting in Chicago in October 2019 and later spoke with the SCGB about his discoveries. An edited version of the conversation follows.
How did you first become interested in studying communication?
About 10% of the population has communication disorders, such as aphasia after stroke or speech symptoms in autism and other disorders. I have both a nephew who is autistic and a father-in-law who had a stroke and lost some of his language ability. Those were both personally moving experiences.
Lack of direct access to the human brain makes it difficult to study the neural circuitry underlying language. How do you get around this?
We know embarrassingly little about the human brain. There is a disconnect between the incredible explosion of methods in neuroscience labs and the still major lack of understanding of how brain cells accomplish something as fundamental as spoken language. The treatments we can use in humans are very crude compared to what we can do in the lab.

Our group is using two different species — the songbird to look at vocal imitation and Alston’s singing mouse to look at vocal turn-taking. We are learning things that really open up our understanding of human speech. We can make predictions based on our work and test them in the human brain.
How are you translating results from birds and mice into an understanding of human language?
In one case, categorizing vocal areas in birds led to a new approach for finding speech areas in humans. About a decade ago, we used focal cooling in songbirds — cooling down an area of its brain — to make the bird sing its song more slowly. This helped to identify a time clock in the bird’s brain. By cooling it down, we slowed the clock, and singing also slowed.
We published a paper a few years ago with our long-time collaborators at the University of Iowa, where we used the same kind of cooling we use in birds to identify speech regions in patients. This is important for neurosurgery — the patient is often awake, and doctors need to map out speech areas so they can leave them unperturbed when removing a tumor or epilepsy-related tissue. Since the 1930s, surgeons have used electrical stimulation to map the parts of the brain involved in speech. But stimulation is like a sledgehammer, potentially leading to errors. It can also cause seizures in epileptic patients. The cooling technique directly targets specific cortical areas with no discernible side effects.
Studying singing mice has also really influenced how we design human experiments. When singing, these mice engage in rapid turn-taking at a speed that resembles human conversation. That inspired us to study the human brain in a different way. We don’t want to just see what happens when a person talks; we want to see what happens when a person has natural vocal exchanges — conversations. This kind of turn-taking is severely affected in conditions like autism.
We are one of the first groups to study the neural basis of vocal interactions by using direct sensors on the surface of the brain to get a high-resolution view of underlying brain activity. Though it seems simple, at the level of speech science it’s almost heretical to study what happens when the subject is engaged in natural conversational exchanges. It has absolutely updated our understanding of how those brain regions work.
Were you surprised by the rapid back-and-forth that takes place among singing mice?
Very surprised. We had been working with them for four years before a rotation student discovered it. He had been spending lots of time with them, trying to figure out how they created their vocalizations. He noticed that animals with cages near each other were chatting incessantly, a behavior known as ‘countersinging.’ Once that was discovered, it broke the whole thing open; that became the story. (For more on Long’s work with singing mice, see “Expanding Neuroscience’s Menagerie of Model Animals.”)
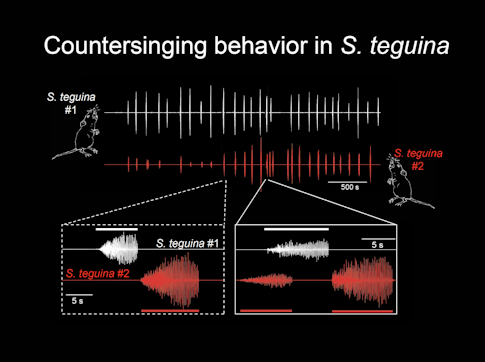
How does this conversational process work in singing mice?
We found that an area in the singing-mouse brain called the orofacial motor cortex (OMC) is essential for rapid vocal turn-taking. If you inactivate the OMC, the mouse can no longer countersing. We think it exerts control over the song pathway, giving the cortex a way to modulate the song, to change its speed, or to stop and start its attempt to communicate with the other animals. We think the notes are structured in some other core area of the brain, but the cortex is able to play that instrument so that two animals can engage with each other.
Have you found neural similarities between human conversation and mice countersinging?
During vocal exchanges, we find a real need for motor planning for both singing mice and humans. There is some functional similarity between the OMC and Broca’s area, a well-known language region in the human brain.
Broca’s area was one of the first brain areas that was ascribed a function — in 1861, French physician Paul Broca met a patient who could understand language but was unable to speak. An autopsy revealed a major lesion in the left hemisphere, the area we now refer to as Broca’s area. Because of a lack of compelling human tools and lack of clear interactive speech paradigms, the field was never able to drill down and study what Broca’s area is really doing.
What have you learned about Broca’s area?
Manipulating Broca’s area causes deficits in coordinated communication. Stimulating Broca’s area causes things like delay and linguistic errors. Both Broca’s area in humans and the OMC in singing mice appear to have a role in coordinated vocal exchange with others, and both show activity related to planning.
Conversation, where one person stops talking and other person picks it up, can happen extremely fast. Other investigators, most notably Stephen Levinson, have shown it takes just 200 milliseconds to transfer conversation from one person to another. It works because Broca’s area is getting ready to engage. The regions that engage speech musculature are ready to go.
How are you going to dig deeper into Broca’s area?
Following in the footsteps of people like Eddie Chang at the University of California, San Francisco, we are now recording with surface electrodes that can give us an idea of what the neural populations in specific cortical areas are doing. We have begun to apply these methods to Broca’s area over the past few years. The dream is to see what’s happening at the level of single neurons and the circuit so that we can connect what we’re seeing in lab experiments to what’s happening in the human brain.
If we find Broca’s area is really important for planning and that process is stymied in certain disorders, we can begin to develop methods to bring back healthy brain function. With more understanding comes more inspiration to develop the next generation of therapeutic tools.
What do you plan to look at next in singing mice?
We’d like to understand how the OMC works, how different signals are routed to different places in the brain. We’d also really like to understand the ethological role of countersinging. We think it has to do with territory. If we learn more about the importance for the animal, we can dig deeper into how the brain can achieve a behavioral goal.
In songbirds, you’re studying how young birds learn songs from their fathers. You’ve found that inhibition is an essential part of the learning process. Why is it so important?
Premotor neurons in the bird’s brain generate neural sequences that allow birds to produce songs in adulthood. When the bird is young, just listening to the father’s song can drive these cells to fire. As birds continue to learn, the father’s song is no longer capable of activating these neurons. The bird is able to block out Dad’s voice, which keeps the song stable once it is learned.
We found that the ability to block auditory inputs comes from local inhibitory interneurons. When we locally turn off that inhibition, we can ‘turn back the clock,’ and those auditory inputs can again drive cells to fire. The process of learning and the change in inhibition happen in parallel as the bird learns the song. Inhibition can selectively protect the notes the bird has learned well, but not the notes it is clumsy with. During construction of the song, inhibition can focus learning on the notes that need the most help. (For more on vocal learning in songbirds, see “Song Learning in Birds Shown to Depend on Recycling of Neural Signals” and “Finding Neural Patterns in the Din.”)
Why would you want to manipulate inhibition?
If I have a perfect song, inhibition acts like a gate that protects the song from change. If we can lift the gate, maybe we can modify a song that had been written in stone. Takao Hensch at Harvard has done similar work in the visual cortex, reopening the critical period for vision and allowing plasticity to work by manipulating inhibition. I think it would be extremely exciting to teach an old bird new tricks. It could help us gain more mastery over the learning process as people age or recover from injury or disease.
First, we need to learn more about what kinds of inhibitory cells are doing this in birds. If we find that this gating is driven by a specific cell population, that will give us a silver bullet to reinvigorate learning in birds. Then we can see if we can do this in other animals.
Do you plan to add other animal models to the experimental menagerie?
We’re considering the budgerigar (parakeet). They are capable of exquisite vocal imitation as well as interactive speech, and both males and females vocalize. What’s exciting about these birds is that they are extremely advanced learners. They can imitate human speech and other complex sounds, so there is a huge possibility space of what they are capable of doing. We just brought some into the lab and are learning about how their brains do what they do.
Is their neuroanatomy similar to that of the zebra finch, the most common bird studied in neuroscience?
It’s completely different. That’s frustrating in the short term but exciting in the long term. These are two very different brain architectures capable of vocal imitation.