
Bang, Bounce or Something Else?
One thing that makes cosmology fun is how much you can notice from simple observations. Why, for example, is the night sky so uniform? If you look in opposite directions to the farthest reach of your vision, the universe looks pretty much the same, yet those distant places have never communicated with each other. They are too far apart. Light from each side of the sky is only now reaching us in the middle, so it hasn’t had time to cross to the other side yet, and no physical process links the two. So why do they look so alike?
That uniformity is a glimpse of a cosmic prehistory. For 13.8 billion years, the universe has been expanding, cooling and evolving. Textbooks often say that the start of this expansion — the Big Bang — was the start of time. But if so, those widely separated regions could never have attained the same temperature and density, and other basic features of the universe would likewise seem inexplicable. “That’s all related to your assumption that there was a beginning of time, so why don’t you give up on that beginning-of-time idea?” says Paul Steinhardt of Princeton University. “That was a simple extrapolation of Einstein’s equations, assuming no change even when you get to energies and temperatures that have never been probed before.”
Most cosmologists think that something must have set the stage for the expansion we observe, although they disagree on what. Steinhardt was a co-author of the standard account — cosmic inflation — but has since turned against it and now promotes a competing model in which our universe is the latest round in a perpetual cycle of creation and destruction. Other scientists, too, are exploring alternatives to standard inflationary theory — and to Einstein’s gravity theory — to fill in the prehistory. “Some alternatives try to provide a pre-inflationary phase,” says Greg Gabadadze of New York University, who is associate director for physics in the foundation’s Mathematics and Physical Sciences division. “Others don’t use the inflationary mechanism but still have a phase which is before the Big Bang — before the conventional expanding phase.”
Cosmologists might finally be approaching some closure on this question. The Simons Observatory, a ground-based array of telescopes designed to make definitive measurements of the cosmic microwave background, is scheduled to see first light in 2020 and reach the requisite sensitivity in five years. Among other things, it will search for gravitational waves from the prehistorical cosmic period, which some models predict and others do not. “Both cases will be interesting: discovery or nondiscovery,” Gabadadze says.
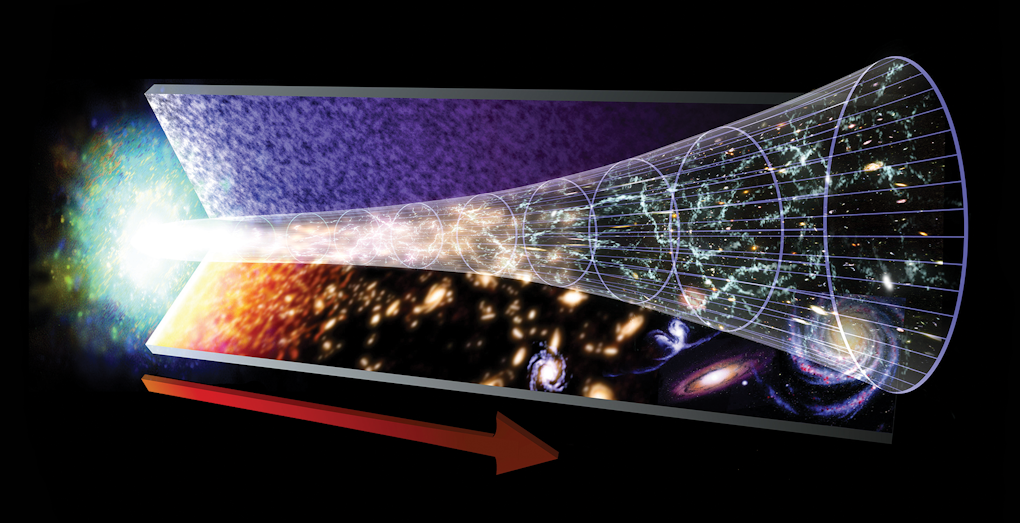
What makes the expansion of the universe tricky to think about is that ‘the’ universe is not the same as our universe. We see only part of the whole, limited by how far light has been able to travel since space began stretching — a distance known as the cosmological horizon. In the standard Big Bang picture, not only does space get bigger, but we see ever more of it. “The rate of stretching is slower than the rate of light propagation through space, so we can receive light from more and more distant sources,” explains Anna Ijjas of the Max Planck Institute for Gravitational Physics.
The ideas for a cosmic prehistory say that one or both of these rates used to differ from what they are now. During cosmic inflation, for example, space expanded at a quickening pace, while the cosmological horizon stayed fixed. An observer during this period would see ever less of space. “It’s the relative growth of space compared to the horizon that’s the important thing,” Steinhardt says. The horizon also limits the range over which physical processes can operate. At first, two nearby regions were able to exchange material and even themselves out. Then space pulled them apart until they exited each other’s horizons, at which point they fell out of touch. Some time later, inflation ended, ushering in the present epoch. Distant regions are uniform because they used to be close together. Similarly, the universe appears to be geometrically flat because the broad curvature of space, if it had curvature, was lost to view.
Steinhardt and Ijjas argue that space need not have grown at all to achieve this effect; on the contrary, it could have shrunk. Cosmologists have toyed with contracting models before, supposing that the universe will reach some peak size, collapse under its own weight back to a pinprick, and bounce to begin a new cycle of expansion and contraction. But those models did not seek to account for cosmic uniformity and flatness. In Steinhardt and Ijjas’ scenario, these attributes are the result of the contracting phase of the preceding cycle.
Moreover, they argue that space need not collapse by all that much before bouncing. Instead it is the cosmological horizon that shrinks to almost nothing. In this scenario, the horizon is defined as how far observers can see before the bounce occurs. “The distance that light can propagate before the bounce gets less and less,” Ijjas says. As the horizon closes in on observers, galaxies vanish from sight and a curtain falls on the broad curvature of space. “Space is becoming more curved in the absolute sense, but your horizon is shrinking faster,” Steinhardt says. “You’re seeing less and less of it, so as you approach the contraction, as far as you can see, that patch looks extremely uniform and flat.”
If you could experience this process, you would lose sight of other galaxies, then stars in our own galaxy, then Mars and the moon, then the other side of the room. Soon your own body would cease to operate as a coherent being, and individual particles would live in utter isolation, no longer interacting. The universe would have completely atomized. All its structures would be frozen in place, emerging from hibernation only when the horizon was able to grow again.
A shrinking horizon is a feature of other alternatives to inflation as well. In so-called Galilean genesis, space initially expanded slowly and had no trouble evening itself out. “At the very beginning the horizon has an infinite size and, as the universe evolved in the genesis phase, the horizon size gets smaller,” Gabadadze says. Additional energy fields and nonlinear interactions among those fields seeded space with matter and revved up its expansion, thereby putting the bang into the Big Bang.
All these models have much in common. They require physics beyond present theories: new forms of energy (akin to dark energy) and perhaps modifications to Einsteinian gravity. They require an even earlier epoch — a pre-prehistory — to make the universe uniform and set up the conditions for expansion or contraction. They naturally produce not just one universe but potentially infinitely many, either in the form of a vast effervescence of bubbles (as in inflation) or as an endlessly repeating cycle (as in Steinhardt and Ijjas’ model).
But the models have crucial differences, too. Inflation occurs when the universe is hot and involves high-energy processes, so it is prone to fluctuations that cause it to produce a huge diversity of universes that vary in the distribution of matter and even some aspects of the laws of physics. Thus, space on its vastest scales is highly nonuniform, which is ironic because cosmologists came up with inflation in large part to explain the uniformity of our observed universe. Our universe is, if anything, a rarity. So the theory fails to make firm predictions for what we should see. Proponents of inflation have sought to tinker with the mechanism or supplement the theory with other principles to give it more explanatory power. In contrast, Steinhardt and Ijjas’ cyclic model avoids this problem because its processes occur at comparatively low energy. It produces universes that are broadly similar, with only minor variations from our own.
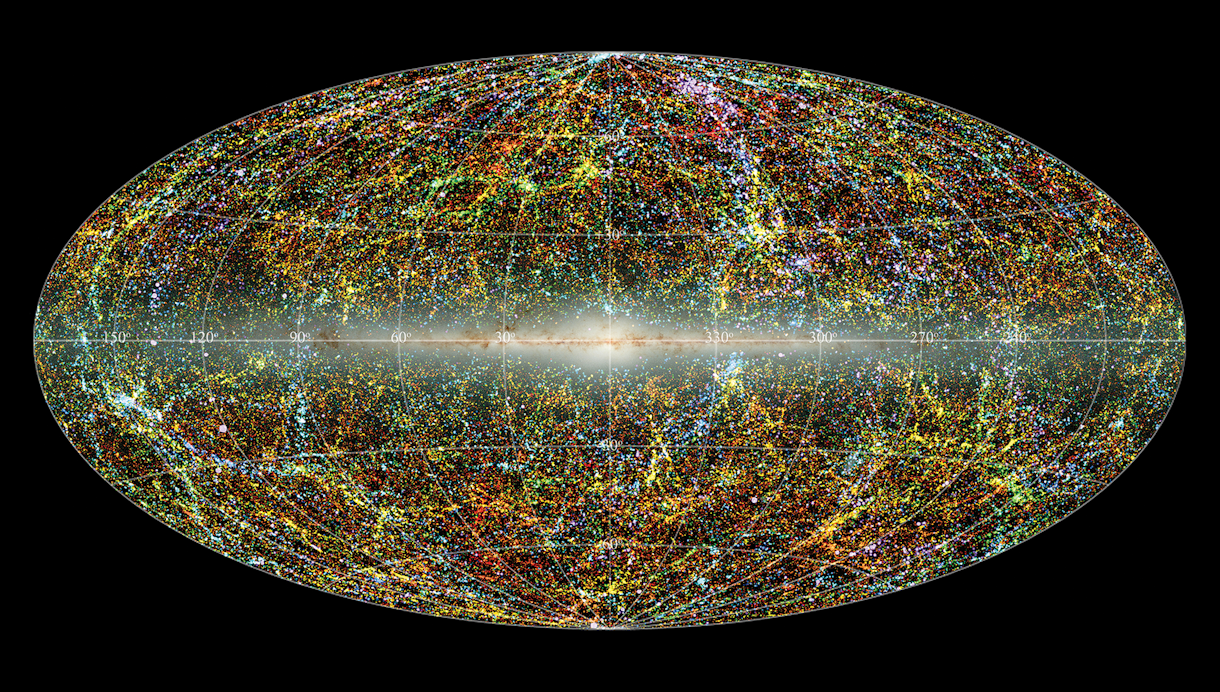
As with many controversies, both sides make a good case, and the task of deciding between them falls to observers. Inflation would have had conspicuous gravitational side effects because it’s a highly energetic process. “When you produce density fluctuations at high energy, they also produce fluctuations in space-time itself,” Steinhardt says. So far, searches for gravitational waves from this era have come up empty. If the Simons Observatory doesn’t find any either, inflation is in trouble. Are the null results to date already uncomfortable? “Yup, they are,” Gabadadze says. “Already they kind of are.”
Conversely, if the observatory does detect primordial gravitational waves, Steinhardt and Ijjas’ cyclic cosmology is dead. “If we see that, it will disprove many of the competing models,” says Simons Observatory director Brian Keating of the University of California, San Diego.
The distribution of background radiation measurements offers another empirical handle on the problem. Currently, a histogram of temperature readings at different locations on the sky traces out a bell curve — a Gaussian. Any deviation from that generic shape would reveal what physics was in play early on. “Primordial non-Gaussianity has to do with the interactions and the number of fields that were involved in inflation,” says Eva Silverstein of Stanford University.
A bounce would require gravitational effects beyond those of Einstein’s theory, and cosmological observations can look for those. “It’s not something that would typically occur, because gravity is attractive, so if you start contracting, you’re going to collide,” says Claudia de Rham of Imperial College London. She and Gabadadze have explored modifications to gravity that not only might let the universe bounce, but would illuminate the mysteries of dark energy. Modified-gravity theories are a steppingstone to a full quantum theory of gravity and, as such, need to satisfy certain general principles. Those principles, along with observations, narrow the range of allowed modifications. “That really constrains your allowed region of parameter space by combining observations and theory priors,” she says.
Once cosmologists open the door to modified gravity, all sorts of new phenomena come rushing in, and bounces are almost the least of it. Frans Pretorius of Princeton, an expert in computer analysis of Einsteinian gravity, has been simulating post-Einsteinian gravity. In one case, he and his students were tracking the formation of black holes when the modified-gravity equations suddenly ceased to operate in time. They had changed their mathematical character from one that evolves to one that remains in a steady state. “When something like this happens, we have no idea how to interpret it,” he says.
As impatient as theorists may be to settle what happened at the dawn of time, or whether time even had a dawn, Keating says his team plans to take it slow. They don’t want to pass judgment before chasing down every possible source of error, not least their own potential confirmation bias, of which any scientist should be acutely aware. “We spend so much time ruminating on what could go wrong,” he says, “we almost need a psychotherapist to help us with our self-doubt.”