The Neural Basis for Number Sense
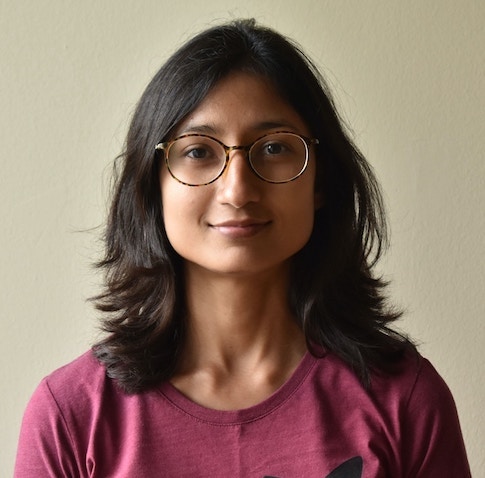
Pooja Viswanathan is an authority on counting — more specifically, what happens in our brains when we count. Why can we easily tell if there are four or five doughnuts left in a box but have more trouble distinguishing 11 from 12 doughnuts left? The difference in both cases is one, after all, but the challenge is much harder with the second group.
Why our brains sometimes struggle with quantities is among the questions that the ever-curious neuroscientist has explored. Now completing a postdoctoral appointment at Rockefeller University, Viswanathan did her doctoral work at the University of Tübingen in Germany and has also studied at the Max Planck Institute and Osmania University. In addition to her expertise on how we process numbers, she is developing new insights into how our brains build social knowledge when we look at people, places and things.
An edited version of our conversation follows.
In your doctoral work, you studied monkeys’ ability to understand the number of objects in a set — what you call numerosity. What do you mean by that term?
Numerosity refers to the number of things there are, or the set size. It answers the question “how many?” In our field, the word means that we showed subjects five colored dots or squares, for instance, instead of the numeral “5.”
We find that different neurons activate upon seeing different sets of dots, so there might be one neuron that gets very activated for sets of two, another for a set of three. But this does not mean that there are separate neurons for every set from one to one million dots. When it comes to large numerosities, neurons get less and less specific about which numerosity they prefer. They might be as activated by 30 dots as they are by 28 dots. Along these same lines, neurons respond similarly to numerosities that are closer together, such as four versus five dots.
We see the same effects in human behavior. We all have a much harder time telling if there are 30 people in a room or 29 people than if there are four or five people, when we can be quite precise. Or we can very quickly tell if there are two beers in the fridge or eight beers, but we might take comparably longer to compare two against three if the beers are sitting close together.
How did you explore numerosity in monkeys?
Monkeys have behavior and brains similar to ours. During my Ph.D., I worked with adult monkeys — not juvenile or infant monkeys — who were not explicitly trained to count numbers. Many researchers, including my Ph.D. advisor Andreas Nieder, had already shown that distinct areas of the brain are activated when monkeys observe and remember the number of items in a set. In these studies, experimenters trained the monkeys to match different sets of numbers and then observed which neurons activated as the monkeys saw various quantities.
I wanted to know whether this number sense arose spontaneously or if it had to be trained. So we designed an experiment that involved no training.
Instead of having monkeys match the numbers in a set, we had them match only the color of the dots and not to count numbers. Even in this case, with no attention to counting numbers at all, different neurons became more active in the presence of different numerosities. We concluded from this that the sense of numerosity develops spontaneously.
What does this work tell us about how humans make sense of the world?
Our work shows that neuronal responses to sets of numbers are not explicitly learned, and they are also not affected by receiving rewards, such as more food, for correctly identifying the number of items in a set.
This work shows that activities in the areas of the brain I studied — the prefrontal cortex and parietal cortex — can be spontaneous and natural, not explicitly learned. These appear to correspond to simple behaviors, which are not goal-directed or relevant, especially in the parietal cortex.
More generally, the spontaneity in how the brain responds to different number sets is what we call the “number sense,” and this is true whether looking at an individual number set or those that are closely related. The neuron that is most active when looking at a set of three, for example, will show some activity when it perceives a set of four.
Do different areas of the brain focus on different aspects of number tasks?
Visual information is first processed in the back of the brain and is then communicated to areas that handle more complex tasks. A lot of neuroscience research in humans has begun to show that information about numbers is processed similarly, traveling from areas that can handle less to greater complexity. For example, when school-going children first learn to count, they show weak neuronal activations in the parietal cortex. When they begin learning about numerals, mathematical symbols and operations, areas that handle more complex tasks, like the prefrontal cortex, become more engaged. It seems that the parietal cortex spontaneously gathers information about the number in a set, and the prefrontal cortex becomes more involved when it is necessary to do something specific with this information. In an experiment I performed in monkeys, I found this progression down to the level of individual neurons in these brain areas.
Your current work at Rockefeller University focuses on how people evolve their social knowledge by looking at other people’s faces, objects or places. How does this work connect with your numerosity research?
How we use numbers changes over time, based on our life experience, and this affects how our brains develop. Someone who seats people in a restaurant, let’s say, probably needs to add things up precisely — and constantly. The rest of us, who rely on calculators whenever we need to count, are likely to have a very different spontaneous counting sense. We are more likely to make rough estimations.
But it is not just numbers. At Rockefeller, I want to understand the granular changes the brain undergoes based on social experience and how much the brain actually changes based on experience. What I would very much like is to capture how our knowledge about those we know is updated as we learn more about them. A lot of these changes occur in areas of the temporal lobe, such as the hippocampus, which I have not explored so far in my career.
How do you plan to study changes to the brain over time?
We will show pictures to monkeys, and as we do so, we will use fMRI data to see the parts of their brains that are especially responsive to faces, compared with objects or other things the monkeys might see. We will then use electrodes to record many neurons in the face-response areas over time. Let’s say a neuron is especially responsive to a particular face — your face, perhaps. We already know from previous research that individual neurons can become very responsive to a particular person’s face, name or voice (the so-called Jennifer Aniston neuron.) How does such a neuron’s response change as the monkey becomes more and more familiar with you? Does the neuron begin to associate what you usually wear or where you might be with your face? How does our perception of faces connect to our perception of places? Oftentimes we cannot recognize someone if we see them out of context, even if we had recognized them correctly many times before. Why is that? I want to find out.