New Map of Brain Vasculature Reveals Unexpected Diversity
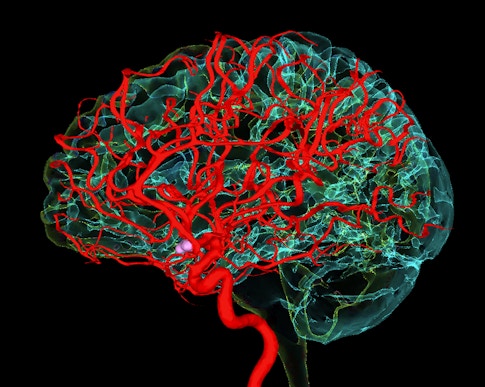
The brain is an oxygen-hungry organ. Consisting largely of electrically active cells that need an energy boost to signal to their neighbors, it consumes 20 percent of the body’s oxygen supply. With no energy stores of its own, it is dependent on a network of blood vessels that work with precision to deliver oxygen and glucose to working neurons at just the right time.
Maintaining this sophisticated transport system, which doubles as the protective blood-brain barrier that keeps toxins and pathogens out of the brain, is critical for both brain function and overall health. Research shows that the brain’s blood vessels deteriorate with age, making us more vulnerable not just to vascular conditions like stroke, but also to neurodegeneration and cognitive decline.
Despite their critical role, the blood vessels that supply the brain remain largely unexplored territory. The cells of the cerebrovasculature haven’t received nearly the attention of neurons or neural immune cells such as glia. Cells that make up this critical interface between body and the brain fall in something of a no-man’s land—not traditionally part of neuroscientists’ domain, but rarely explored by researchers focused on the development and function of blood vessels, either, says Lee Rubin, a neuroscientist at Harvard University and an Investigator with the Simons Collaboration on Plasticity and the Aging Brain. But that’s beginning to change.
Early in 2022, three research groups independently described the first molecular atlases of the cells that make up the vasculature of the human brain. By profiling gene expression cell by cell, they have identified new cellular subtypes and detected the expression patterns of known disease-associated genes, suggesting that vascular changes may be key to the pathology of Alzheimer’s and Huntington’s disease. The new cellular censuses are expected to help researchers explore how molecular changes to the vasculature disrupt brain function, and how targeting cells of the vasculature might help prevent cognitive decline and other brain dysfunction.
The findings are of particular interest to researchers studying brain aging. The brain’s vasculature deteriorates as we age, becoming both less dense and less functional, reducing blood flow to brain cells. Moreover, a growing body of research shows that factors in the blood can rejuvenate the aging brain, but researchers don’t know precisely how. A detailed map of the brain’s vasculature will provide researchers a better baseline for investigating precisely how it changes with age. It may also help explain how factors carried by the blood can rejuvenate an aging brain and perhaps hint at ways to enhance them. (For more, see “Searching for the Secret of How Young Blood Rejuvenates the Brain.”)
Tony Wyss-Coray, a Stanford neuroscientist and SCPAB investigator who led the development of one of the new atlases, hopes the findings will open a new frontier in neuroscience research. “It was always surprising to me that people initially focused only on neurons,” he says. “There was zero interest in other cell types.” For a long time, genetic mutations linked to neurodegenerative disease were assumed to be directly responsible for neuronal disfunction, he says. That’s changed in recent years, as the brain’s resident immune cells, the glia, have risen to prominence. But that view is still too narrow, Wyss-Coray says. “We hope with this paper we tell people … there’s actually a lot of other cells in the brain.”

A collaborative cellular community
Keeping the brain supplied with blood-borne nutrients while protecting it against harmful molecules requires the coordinated activity of a diverse set of cells. Many of these are similar to the cells that comprise blood vessels in other parts of the body. But due to their unique relationship with the neurons they support, they have identities all their own. The endothelial cells that line blood vessels in the brain are closely associated with fibroblasts that provide structure, muscle cells that regulate blood flow, pericytes that mediate interactions with neurons, and astrocytes and other immune cells that help maintain the blood-brain barrier.
Though the blood-brain barrier is often thought of as a barely penetrable partition, Wyss-Coray has found that many proteins pass through blood vessel walls and enter the brain. In research published in 2020, his team found a substantial amount of protein uptake in the brains of mice. Uptake of blood-borne proteins dropped by half in older animals, reflecting an age-related shift in the way molecules are transported across blood vessel walls. As animals age, the proteins needed to ferry specific proteins into the brain decline. At the same time, a less selective form of transport becomes increasingly common, permitting molecules that were previously excluded from the brain to traverse the barrier.
The age-associated loss of blood vessels’ barrier properties is likely exacerbated by a number of factors, such as loss of the latchlike proteins that seal the space between adjacent endothelial cells. Decline of the barrier accompanies other age-related changes to the cerebrovasculature. Structural changes observed in aging animals include a loss of capillary density, stiffening of artery walls and increased tortuosity in small vessels. Blood flow may become less tightly coordinated with neural activity with age, as well.
Many neuroscientists suspect that changes like these — whether due to normal aging or through the early stages of neurodegenerative disease — set the stage for more severe loss of function in the brain. “A common feature across Alzheimer’s disease, Huntington’s disease, ALS and other age-related neurodegenerative diseases is that blood-brain barrier dysfunction and cerebrovascular abnormalities often precede more overt disease-specific symptoms, often by decades,” says Myriam Heiman, a neuroscientist at MIT. “If we could understand the basis of this, this could potentially be a way of delaying or inhibiting some disease processes generally, across the age-related diseases.”
Mapping the frontier
Most research on brain vasculature has focused on mice. While that work has been informative, significant differences exist between mice and humans. “The complexity of the vasculature — density, the amount of wrapping by glial cells, the accessory cells — scales with complexity of the nervous system,” Heiman says. That has consequences for understanding human aging and disease, as well as for therapeutics: Many experimental compounds tested in animal models fail clinically because they can’t make it across the blood-brain barrier in patients, for example.
Creating a cellular resolution map of human brain vasculature has been challenging for several reasons. Vascular cells are so outnumbered by neurons and glia that they have been largely overlooked in brainwide analyses. They’re also enmeshed in a stringy matrix that makes it difficult to separate them from one another. And while the nuclei of certain types of vascular cells may be preserved when intact brain tissue is prepared for single-cell RNA sequencing, others are likely to be destroyed or left behind, Wyss-Coray says.
To overcome these hurdles, Wyss-Coray’s group, and a group led by Heiman and MIT computational biologist Manolis Kellis devised methods for isolating nuclei from the complex mix of cells that make up the vasculature from precious postmortem brain samples. Heiman and Kellis also included tissue removed from patients’ brains during neurosurgery at Children’s Hospital Boston, rushing the samples back to the lab and processing them within the hour. All told, the two groups amassed more than 150,000 nuclei for single-cell analysis, from both healthy brains and brains affected by neurodegenerative disease.
Using RNA sequencing, the teams generated complete gene expression profiles for each cell. Heiman and Kellis compared the brains of healthy individuals with those of people with Huntington’s disease, while Wyss-Coray looked for differences between healthy brains and brains affected by Alzheimer’s disease. Both studies were published in Nature in February. A third group, led by neuroscientist Tomasz Nowakowski at the University of California, San Francisco, conducted a similar analysis, using single-cell transcriptomes to compare the cellular composition of healthy human cerebrovasculature with that found in abnormal tangles of blood vessels called arteriovenous malformations, a rare condition that increases the risk of stroke. The teams’ analyses of healthy brains uncovered unexpected cellular diversity, including several notable surprises.
Wyss-Coray and Heiman and Kellis’ groups both found that based on divergent patterns of gene expression, the brain’s pericytes, which form much of the interface between the blood and the brain, can be separated into two subtypes. Wyss-Coray says one group looks to be specialized for transporting materials across the blood-brain barrier, whereas the other may be dedicated to building and maintaining the matrix that surrounds the cells and constitutes the bulk of vessel walls. Likewise, the new atlases provide the first characterization of fibroblasts, a type of cell that generates extracellular matrix, within the human brain. According to Heiman and Kellis, genetic activity within cell fate pathways suggests some of the brain’s fibroblasts may be able to differentiate into pericytes, echoing observations that others have made in the brains of zebra fish.

One of the key lessons emerging from these analyses are the significant differences between mice and humans, which could be important as researchers work to understand disease. “Gene expression is not as well conserved between mouse brains and human brains as we had anticipated,” says Wyss-Coray. He notes that some genes that have been studied in the mouse vasculature, and even used as cell type markers, were active in different cell types — and in some cases not active at all — in the human vasculature. “That’s a big concern if you are trying to understand the function of the vasculature or making inferences about the possible role of genes in disease,” he says. Researchers also found that the genes whose expression patterns vary along the different parts of blood vessels, a phenomenon known as “zonation,” differ between mice and humans. Because of these disparities, Wyss-Coray, Heiman and Kellis agree that cultured human cells and tissues, including stem cell-derived models, will likely be necessary to fully understand the vasculature’s role in human aging and disease.
Exploring aging and disease
Researchers hope their findings will draw attention to the diversity of cells comprising the cerebrovasculature and how these cells contribute to brain function. Both the Wyss-Coray and the Heiman and Kellis studies found that genes previously linked to disease were active in these cells, and that expression patterns differed between healthy individuals and those with Alzheimer’s disease or Huntington’s disease. Understanding the role of brain vasculature in disease will be important both for understanding the origins of neurodegenerative diseases and for developing new therapeutics, Heiman says, given that blood vessels are more accessible than the neurons that they support and protect. “Therapeutics delivered to the blood can access the endothelial cells of the brain,” she says. “So now that we have a list of things that seem to be dysregulated in Huntington’s disease, there are many interesting directions, from gene therapy to perhaps pharmacological studies.”
Likewise, with the molecular composition of the cerebrovasculature of healthy brains now carefully mapped, researchers can begin to investigate the age-related changes that accompany cognitive decline. A detailed map of the cerebrovasculature’s different cell types will make it easier to track how gene expression and cell function change with age — and to search for ways to reverse these changes.
In Rubin’s lab at Harvard, for example, researchers are exploring ways to restore full function and growth potential to aging vasculature, which will require understanding and targeting the right cell types. While endothelial cells don’t usually proliferate in adult brains, they can: Their growth is essential to giving rise to new blood vessels after stroke or traumatic brain injury. Rubin explains that his group’s own single-cell analyses have identified a subpopulation of endothelial cells that express genes associated with mitogenesis and proliferation. “They’re not mobilized, but it suggests the potential for targeting subpopulations of endothelial cells,” he says. “It’s early days, but it seems sort of intriguing to me that this would be an interesting and important cell to work on.”