What the Study of HIV Can Tell Us About the Brain

During his doctoral research, first year Simons Junior Fellow Benem-Orom Davids discovered new details about how HIV exploits one of the body’s immune defenses to infiltrate its host’s DNA. This irreversible step of the virus’s life cycle, called integration, works by rendering the virus a permanent part of the host cell, allowing the infection to persist and spread.
Today we have several effective drugs that can manage HIV infection, transforming it from a death sentence into a chronic condition. Davids’ doctoral work on the mechanisms of integration points to the importance of understanding the interactions between viruses and cellular host factors, which is critical for developing drugs that thwart HIV’s ability to replicate — and would get us closer to an actual cure for HIV.
Davids, who earned a bachelor’s degree in biology at Morehouse College and a doctorate in biochemistry from Meharry Medical College, is now a postdoctoral fellow in microbiologist Stephen Goff’s lab at Columbia University Irving Medical Center. At Columbia, Davids is studying whether there’s a viral component to how we remember new information.
Davids and I recently discussed his work on HIV, and how it has informed his new focus on neuroscience. Our conversation has been edited for clarity.
At Meharry Medical College, you identified new information about the role that the protein TREX1 has in making HIV such a successful virus. What did you uncover?
Just like grocery stores have specific aisles for displaying different goods — frozen foods, paper goods, canned items and so on — our cells have compartments dedicated to specific tasks. For example, our DNA is housed in the cell’s nucleus. This keeps it separate from the surrounding cytosol, where our cellular defenses work to recognize and remove foreign material, such as viral DNA, that can sometimes sneak in. When this so-called cytosolic DNA activates the cell’s defenses, special proteins known as nucleases degrade and flush out the invaders. TREX1 is one of these nucleases.
However, HIV can co-opt TREX1’s normal duties to enhance infection. This is because TREX1 preferentially degrades ‘unprocessed’ viral DNA, which happens to be incompatible with integration. In other words, TREX1 focuses on removing viral DNA that wouldn’t have harmed the cell anyway. This has the unfortunate effect of increasing the proportion of viral DNA in the cell that is capable of integration. Over time, this drives HIV infection. We published this work in the Journal of Virology in 2021.
Before this discovery, scientists believed that TREX-1 helped drive HIV infection by helping the viral DNA evade detection by the innate immune system. Now we know that it plays a more proactive role, by making it easier for viral DNA to integrate into genomic DNA in the nucleus.
What an unexpected — and important — discovery! Are there still open questions as to how HIV works?
Absolutely. One key question relates to histones. These are proteins that provide structural support to chromosomes, like a kind of molecular scaffolding. Scientists are working to understand whether marks on a histone can show HIV where it can integrate. This work could help inform the development of drugs that target these marks as way to slow integration.
Another open question is related to HIV latency. It has long been observed that, after the initial infection, HIV goes silent for long periods of time before it’s suddenly active again. We’re not sure why this happens, but it has been one of the key barriers to finding a cure. This is because, antiretroviral drugs only work when the virus is active, and have little effect on any latent HIV reservoirs. Solving the mystery of this latency is critical to finding a cure for HIV.
Let’s turn to your current postdoctoral research, which involves something called endogenous retroviruses (ERVs). What are those, and why are they important?
Let’s take the definition in two parts. A retrovirus is a type of virus that inserts a copy of its genomic material into the DNA of a host cell that it enters, thereby becoming a permanent part of the host cell’s genome. HIV is a classic example of a retrovirus. And ‘endogenous’ simply means present in the germ-line DNA of the living organism, such as an animal or a human.
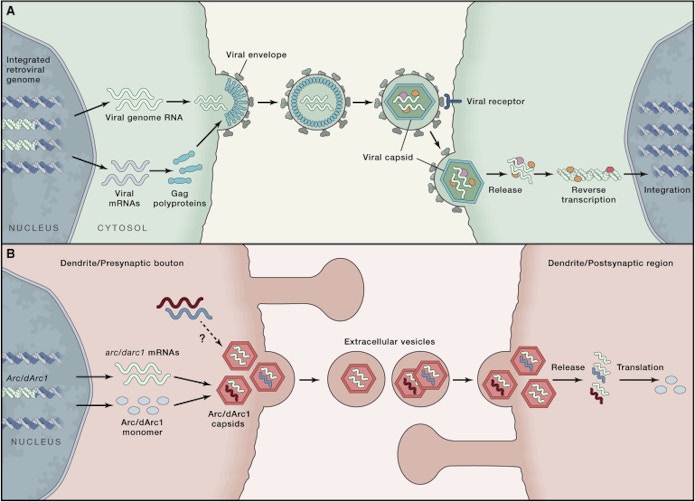
Approximately eight percent of our genomes are made up of viral sequences. These are the remnants of viral infections that integrated into our germ line millions of years ago. It is possible that TREX1 assisted with some of these ancient integration events.
Most human endogenous retroviruses (hERVs) have lost any pathogenic elements that they once had, and today some play important roles in our bodies. For example, a protein involved in the function of the placenta, which nurtures a developing fetus, is derived from the envelope protein of an ERV. Yet another fascinating example is the Arc protein, which plays an indispensable role in how the brain remembers, and is well-known in the field of neuroscience.
Arc is derived from an ancient viral Gag gene and is structurally similar to the protein by the same name found in HIV. Recently it has been suggested that Arc may have retained some of its virus-like functions in the brain. Here at Columbia, we are eager to characterize the potential Gag-like properties of the Arc protein, and are particularly excited to find out if these functions influence Arc’s role in the brain. So is there a viral component to how we remember? It seems like it, but that’s what we are trying to find out.
What do we know about Arc so far? And what are you discovering?
Great question! The Arc protein is so fascinating because it does so many things. Originally, researchers noticed that Arc levels in the brain increased following a spike in neuronal activity. Later, it became known in relation to synaptic plasticity — a key process believed to be involved in forming and solidifying memories. We are also intrigued by the recent finding that suggest Arc can self-assemble into a structure known as a capsid that envelopes RNA — a phenomenon that is also a hallmark of retroviruses like HIV. The exciting idea we’re now exploring is that Arc is mediating the transfer of mRNAs across synapses, from a donor cell to a recipient cell, perhaps in response to neuronal activity, and thereby modifying the characteristics of the synapse.
This is what we know about Arc so far. But we don’t yet know what drives Arc to self-assemble, or what RNA is packaged inside the capsid. We’re looking at these and other questions, with a focus right now on how Arc is released from cells and on understanding the requirements that drive RNA packaging into Arc capsids.
While there is much yet to discover, the exciting big-picture point is that our brain activity may depend to some extent on virus-like activity; if that is the case, this would be a novel discovery. I am fortunate to be able to study these questions with Steve Goff, a leading authority on retroviruses.
Finally, what are your thoughts about the Simons Junior Fellowship?
Networking with the other Junior Fellows who are training in the city, especially during our weekly dinners, is not only a source of potential collaborations but also an opportunity to think about my work from new perspectives.
When I describe my work to a physicist, they’ll ask different questions than the biologists I usually speak to, and vice versa. I hope my questions spur the other Junior Fellows to think about their work in a new way as well.