
Computational Biology Goes Under the Microscope
In a dark room, developmental biologist Jasmin Imran Alsous slides a sample under a large microscope. On a nearby computer screen, a tiny world appears. Long, colourful threads dance across the display. Labeled through genetically encoded fluorescent tags, these bright red and green filaments are the heads and tails of fruit fly sperm.
Measuring a whopping two millimeters in length, fruit fly sperm are a biological oddity. Nearly as long as the fruit fly itself, these sperm manage to propel themselves past each other while remaining untangled inside the organ where they are stored. Figuring out how they move without becoming a knotted mess is one focus of Imran Alsous’ research.
Imran Alsous’ work, which is centered on constructing computational models of these and other biological phenomena based on experimental data, has been transformed by a new facility at the Flatiron Institute’s Center for Computational Biology (CCB). Known as the CCBScope Observatory and consisting of a state-of-the-art microscope, its goal is to more fully enjoin theoretical and experimental biology by enhancing computational modeling with dedicated experimental resources. This synergy will aid in the development of theoretical models of fundamental biology and microscopic processes, including Imran Alsous’ models of fruit fly sperm.
Launched in July 2022, the CCBScope Observatory is the only experimental venue at the Flatiron Institute, which was founded in 2016 in New York City to advance cutting-edge scientific computational research. CCB scientists are focused on computational and theoretical modeling of biological problems whose scale and complexity have typically defied analysis, and usually rely on experimental data from other scientists to advance their work.
The new project was spearheaded by Flatiron scientists Reza Farhadifar and Imran Alsous, both research scientists at the CCB. By allowing Flatiron scientists to generate and control their own experimental datasets, the CCBScope Observatory is speeding up the research process by directly involving theoreticians in decisions connected to data collection and analysis, and allowing theory and empirical studies to advance in tandem and iteratively.
“Having our own setup allows us to very systematically collect data,” Imran Alsous says. “It is now possible to become less reliant on other datasets that are combined from multiple studies, often under different experimental conditions.”
The combination of experimental and computational research Imran Alsous, Farhadifar and their CCB colleagues are conducting with the CCBScope Observatory is already advancing fundamental biology, helping scientists better understand how cells move and organize themselves, and making breakthroughs that could potentially have applications in medical sciences down the road.
Simplifying the research process
Unlike the defined laws that govern the physical realm, biology is messy. When modeling biological systems, researchers depend heavily on experimental data. In order to refine their models to accurately represent fundamental phenomena, theoreticians have to continually go back and forth between real systems and the theoretical models they’re developing.
“Biological modeling is complicated because there are so many moving parts,” says Michael Shelley, applied mathematician and CCB director. “It has to be very carefully guided by experiment.”
Theoretical biologists, including those at the CCB, typically work with second-hand experimental datasets. These datasets are created by experimental biologists working on their own specific projects. As a result, the data the theoreticians have access to is not always ideal for their specific applications and sometimes has to be piecemealed together from different sources. Even at universities and institutions where theorists are able to work closely with their experimental colleagues, there often remains a gap between what data they need and what they can get. The CCBScope Observatory was imagined as a solution to this problem. By having an in-house microscope facility, the CCB’s scientists now have the ability to create datasets specifically for their own purposes.
“This project is an opportunity to build an intimate collaboration between experiment and theory. This is not the typical experiment where theorists watch from a distance,” says Shelley. “We are building bridges between theory and experiment in cell biology.”
The CCB is primarily a theoretical institute, but several scientists came here with experimental backgrounds and experience from previous research positions, which helped make CCBScope Observatory possible. This includes Farhadifar and Imran Alsous, who have both worked on research projects that were heavily dependent on gathering experimental data and using theoretical models to rationalize the data and to make predictions that motivate future experiments.
“When we began thinking about the project, we realized we already had all the expertise at the Flatiron Institute, waiting to be leveraged. We have experts at fluid mechanics, we have experts at machine learning, and we have experts in polymer biophysics, all under one roof,” Imran Alsous says. “Already the collaborations we have underway through the CCBScope Observatory feel seamless.”
Leveraging advances in technology
The initial planning for the CCBScope Observatory began in early 2021 and it took over a year for the project to fully come together. First, two rooms at the Flatiron Institute designated for the CCBScope Observatory were cleared and prepped for their new purpose. In the room housing the microscope, windows were darkened, airflow was controlled, and temperature was adjusted. Another room was prepared for sample prep, and old equipment removed. Specialized equipment and samples were ordered. A comprehensive safety plan was created with help from Simons Foundation vice president of administration, Marion Greenup. In the summer of 2022, the new microscope arrived from Japan.
The CCB’s microscope is an oven-sized giant with a vague resemblance to the arcing microscopes used in grade schools. The instrument is a laser-scanning confocal microscope that can capture tens of images per second at sub-micron resolution. Sitting atop a specialized table that reduces vibrations, the CCB’s microscope is connected to a computer that allows the scientists to capture images and high-speed videos of their samples.
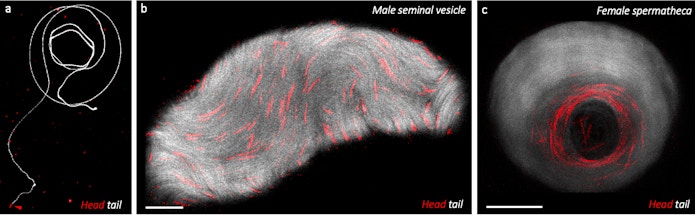
“We can image in four different colors at the same time,” says Farhadifar. “This means that we can see multiple fluorescently labeled proteins in one image, which allows us to investigate how they interact.”
The new instrument is already allowing scientists to see processes that were impossible to capture even five years ago by taking wide field of view images in three dimensions as well as record video with up to 100 frames per second. But more important than the technological advancement is the ready access the CCBScope Observatory offers to scientists. Being able to take as many images as they need helps the scientists reduce uncertainty in their measurements and models. The researchers are also able to test the predictions of their models and make further refinements; after all, the theoreticians are literally next door.
Back to the basics
The CCBScope Observatory is currently set up to study two organisms: fruit flies and Caenorhabditis elegans (C. elegans), a type of small roundworm commonly known as a nematode. These so-called ‘model organisms’ are often used in experimental studies because they are relatively simple (especially when compared to mammals), they reproduce quickly, and they are easy to work with because of all the tools that the broad scientific community has developed for their use.
“Organisms like nematodes are useful because they are very old evolutionarily,” says Shelley. “And humans actually share an enormous amount of the same machinery.”
Farhadifar is one of the scientists working with nematodes. He uses the tiny organisms to study cell division. Cell division begins shortly after fertilization of an egg cell and occurs constantly in our bodies as we grow up and grow old. During our lifetimes, our cells will divide a staggering 10 quadrillion times.
“People have been studying cell division for nearly two centuries now,” Farhadifar says. “But even after so many years, we still don’t understand a lot of the details.
Farhadifar focuses on the first instance of a cell dividing right after fertilization, when it is easiest to see. In the past, Farhadifar has used this moment to develop simple models of spindles — an integral structure that forms in a cell during the division process. With the CCBScope, Farhadifar is digging further into the details to understand how proteins are involved in creating forces that cause spindle movement.
The proteins Farhadifar is investigating are found in nearly every form of life from yeast to humans. This universality makes it possible to extend the findings from nematodes to other organisms. Already Farhadifar has used his nematode-based spindle model to successfully explain behaviors in human tissue cells. The hope is any findings with proteins will also be universal.
“The questions we’re investigating are essential for understanding basic biological processes,” says Farhadifar. “Furthermore, we need to understand these biological processes because they are highly related to diseases.”
By understanding how cell division works, scientists can better understand what happens when things go awry. When cells divide too quickly for example, it can result in cancer. In fact, a common chemotherapy drug, Taxol, works to slow cell division by inhibiting the formation of microtubules — proteins in cells that help segregate genetic materials during mitosis, the process where chromosomes replicate. By studying fundamental cellular processes, Farhadifar hopes the discoveries they make could someday lead to similar advances in medical sciences.
While the CCBScope Observatory is just getting underway, those involved are excited by the possibilities the new project is bringing to the CCB. From understanding how fluids flow in cells to mysteries of gamete movement, the CCBScope Observatory will enable new discoveries about fundamental biology in the years to come.
“In five years, I hope we really have a comprehensive picture of this one model organism, how it moves into its first cell division,” says Shelley. “I hope that from this effort we will have developed a comprehensive model, like a so-called ‘grand unified theory’, of the processes that initiate cell division. I hope we will be able to understand it in a very intimate and elegant way.”