How Do Our Neurons Change When We Encounter New Things?
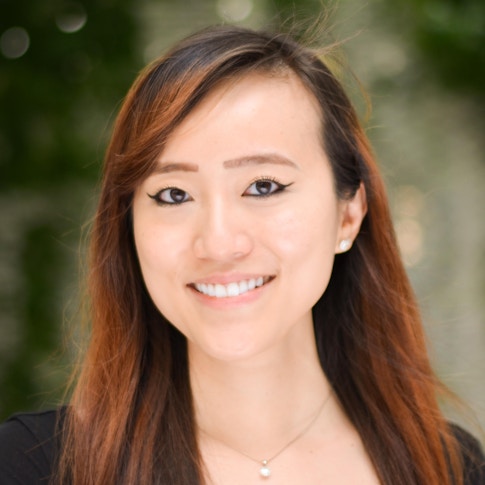
Lynn Yap is fascinated by how we learn new things. Sometimes our minds get used to patterns, which can prevent us from thinking ‘outside the box.’ But at other times we can easily adapt, forming new, creative answers to unfamiliar questions. Yap herself epitomizes this ability to adapt; still early in her career, she has pivoted from a focus on chemistry to a current emphasis on neuroscience.
Now a second-year Junior Fellow with the Simons Society of Fellows, Yap is completing a postdoctoral fellowship in the lab of Nobel Laureate Richard Axel at Columbia University’s Zuckerman Institute. She holds a doctorate in neurobiology from Harvard Medical School and a bachelor’s degree in chemistry and biology from Yale University.
We recently discussed Yap’s current work and scientific path. Our conversation has been edited for clarity.
What led to your interest in the neurobiology of learning and memory?
When I was 12 or 13, I remember feeling a ‘spark’ during chemistry class. I watched our teacher draw the components of an atom on a chalkboard: protons and neutrons in the center, electrons orbiting around the nucleus. Learning that there existed particles smaller than an atom ignited a deep curiosity in me.
It led me to study chemistry in college, where I learned to develop small-molecule drugs under the guidance of many invaluable mentors. Toward the end of college, I came to realize that a better understanding of a disease’s underlying biology would make me a more effective chemist. After graduating from Yale, I joined the lab of the late Paul Greengard, Nobel Laureate at the Rockefeller University. The lab studied neurodegenerative disorders, such as Alzheimer’s and Parkinson’s diseases, using rodents as animal models.
A major feature of both of these diseases is memory impairment. This was when I first learned that scientists could use small animal models to study the neurobiology of complex processes such as learning and memory. I have studied learning and memory from various angles ever since.
Why are small animal models important to this work?
Animal models such as mice have similar genomes to ours, and so the pathophysiology of various diseases can be quite similar. For the work that I do, mouse models are necessary because we need to record the activity of hundreds of neurons across various brain regions at a cell-by-cell level, something that we of course cannot do easily in humans. On top of that, wherever possible we also manipulate these neurons to better understand cause and effect, and to do so we need genetically modified strains and molecular tools only available in mice.
Can we extrapolate results in mice to gain insights about people?
We have gained a lot of insights from studies in mice that scientists have been able to extrapolate to humans, especially from a molecular and cell biological perspective. When studying cognition in mice, it should be noted that our animal subjects cannot report to us what they are thinking, remembering, learning, or feeling. While we can only infer these cognitive processes based on their behavioral actions, they remain a critical tool for informing research on human psychiatry and in drug development.
What research did you undertake during your doctorate at Harvard Medical School?
My doctoral research focused on neural plasticity, which is the brain’s ability to respond to a detectable change in the environment. Neural plasticity is the reason we can learn new things.
I completed my research in Michael Greenberg’s lab at Harvard Medical School. We sought to better understand neural plasticity from a genomic, molecular and synaptic perspective.
When a neuron fires substantially above its baseline rate — for example, when something novel is happening — a gene known as Fos is transiently expressed. Almost 40 years ago, Dr. Greenberg discovered that Fos is induced in response to neuronal activity. Since then, Fos has been widely used by the neuroscience community as a tool to identify and mark active neurons.
A lesser-appreciated fact about Fos is that it is a master regulator, which means that when expressed, it goes on to orchestrate the expression of hundreds of other genes, many of which are critical for plasticity. We still understand very little about what types of plasticity Fos drives, how Fos drives these changes and why these changes matter. During my Ph.D., I tackled these questions in the hippocampus — a brain region responsible for learning and memory.
I described how different inhibitory neuron subtypes undergo different types of synaptic changes as animals encounter novel environments. Using a variety of genomic approaches, I discovered that Fos controls the expression of another gene called Scg2 — which facilitates communication between neurons.
In separate but related work, myself and collaborators from Christopher Harvey’s lab at Harvard looked at how Fos-expressing neurons in the hippocampus responded as mice entered specific places. We found that Fos cells encoded location much more stably from day to day compared to cells that did not express Fos. Thus, Fos seems to be driving the type of neural plasticity critical for the long-term memory of places. This is how we can call up a mental image of our elementary school, even if we have not seen it in years.
We published this work in Nature in 2020 and 2022.
What are you working on since arriving at Columbia?
For my postdoctoral work, I have returned to the world of chemistry, in a sense. I am now trying to understand how mice learn about the volatile chemicals, or odors, in their environment. The myriad of sensory stimuli we encounter in our environment typically lack immediate significance — it is through experience that they are afforded meaning. One focus of my research is in understanding how the value of olfactory sensory stimuli is learned over time.
The other aspect of my postdoctoral work focuses on how animals learn about the statistical regularities of their environment. More specifically: How do neurons respond when they have repeatedly encountered the same set or sequences of stimuli, and how do they know when there is a change in this landscape?
These questions are at the heart of learning and plasticity, and I am employing the olfactory system to study them.
I gain inspiration from eminent scientists before me who have looked at this problem from a different perspective: that of spatial navigation, which seems to work similarly in animals and humans.
Let’s say you have a road trip planned for the weekend. If it’s a trip you’ve taken many times before, you can probably map out the route in your head, without needing to consult a GPS. It turns out that rodents tend to operate the same way; they also keep internal maps of their spatial environments.
Given that these animals primarily rely on their sense of smell to do just about anything, when we look at areas that are responsible for processing odors in the mouse brain, we’re looking for evidence that they also form internal maps or expectations of learned odors. Can they detect when something is amiss — for example, when an expected odor does not appear, or when an unexpected odor appears in an otherwise familiar environment? How does this ‘mismatch’ get computed in the brain, and what does the brain do with this information? How does the brain then use this ‘error’ it detected to update its current understanding or expectations? Those are the questions I am asking today.
Finally, what are your thoughts about the Simons Junior Fellowship?
I am very grateful for the community of Junior Fellows. When entering graduate school, you usually begin with a cohort of classmates with whom you take the same classes and experience the joys and challenges of completing your Ph.D. Every Ph.D. journey is unique, yet you have peers that are going through similar experiences and can relate at a deep level.
Being a postdoctoral fellow can be a bit of a jarring experience at first; you typically don’t enter with a cohort of other fellows, so it can be hard to find your footing. Our community, whose members span different institutions but are all based in New York, provides this much-needed opportunity to connect with, and learn from, like-minded people.