Experiments and Mathematical Model Uncover the Mechanisms Behind Wrinkles in Cell Nuclei
With age, comes wrinkling. This is true even for the tiny organelles in our cells that carry our DNA. As these organelles — called nuclei — age, their once-smooth surface forms divots and folds. This ‘wrinkling’ has been linked to aging and disease. However, understanding how wrinkles form, and the biophysical processes that cause them, has long proved challenging to scientists. Now, a new study co-led by a researcher at the Flatiron Institute in New York City has revealed the basic principles of nuclear wrinkling. The results not only determined the unique characteristics of the wrinkles — which were surprisingly consistent over time — but also provided insight into how that wrinkling can be controlled.
These findings, observed in the nuclei of fruit fly cells, are a critical step toward deciphering the interplay of genes and geometry in cell nuclei. The study was published in the journal Nature Physics in September.
“We saw these nuclei wrinkle like crumpled paper, which was surprising because we typically think of membranes as behaving more fluid-like,” says Jasmin Imran Alsous, project lead and developmental biologist at the Flatiron Institute’s Center for Computational Biology, who was a corresponding author on the study.
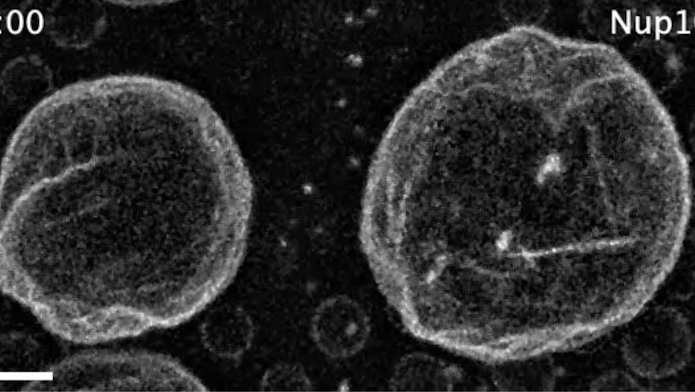
By clicking to watch this video, you agree to our privacy policy.
The study specifically looked at a fruit fly’s nurse cells. These cells are connected to an immature egg cell — an oocyte — and are responsible for helping that oocyte grow. In each fruit fly egg chamber, fifteen of these nurse cells pass an immense amount of proteins, mRNA and other nutrients to the oocyte via tiny bridges; they ultimately die, and the oocyte remains.
“I think of the oocyte as a queen bee and the nurse cells as worker bees,” Imran Alsous says.
To deliver so many nutrients, each nurse cell grows to more than ten times its original size in a period of just three days. This growth is accompanied by the appearance of folds and deformations in the nuclear envelope, making these cells ideal testbeds for studying the mechanisms of wrinkling.
“We were working on a different project related to transport of material from the nurse cells to the oocyte and happened to notice these nuclei becoming very visibly wrinkled, and that effect was highly reproducible,” Imran Alsous says. “Because the nuclei in these cells are so large, it makes it very easy to see and study. Almost everyone can visualize what a wrinkled surface looks like, so we thought this would be a great and relevant problem for us to try to figure out.”
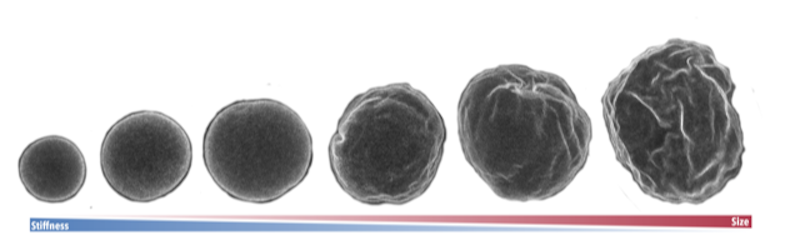
The scientists first set out to characterize the wrinkles by taking high-resolution, three-dimensional images of nurse cells in their home egg chambers at various points spanning approximately thirty hours of development. They relied solely on live imaging of nuclei whose membranes were fluorescently tagged. Importantly, live imaging allowed them to directly observe the wrinkles as they appeared and disappeared. These experiments showed that the wrinkles were highly dynamic, constantly changing and fluctuating.
“It is rare to have such exceptional 3D imaging data, in terms of quality and quantity, and it allowed us to pin down specific biophysical properties of the cell nuclei that are difficult to access otherwise,” says study co-author and co-principal investigator Jörn Dunkel, a professor of mathematics at the Massachusetts Institute of Technology (MIT). “More importantly, these data put us in a position to make and validate specific predictions for how to control the surface deformations of cell nuclei.”
With images of over 300 cells of various sizes in hand, the group set out to quantify the wrinkles and characterize their dynamics. Biological physicist Nicolas Romeo, who performed this research while at MIT and is now a postdoctoral fellow at the University of Chicago, used a mathematical tool called spectral analysis that could map the shape of the nuclear surfaces. The technique has previously been used for studying other systems that are close to spherical, such as weather patterns on planets and even the cosmic microwave background.
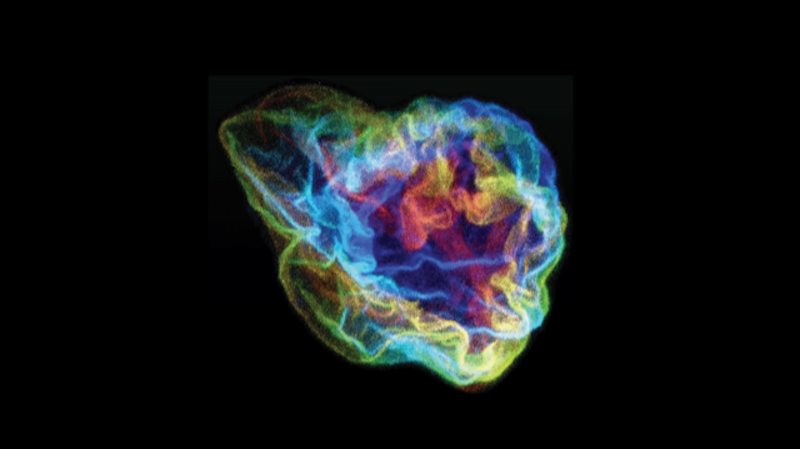
“There is a whole language of mathematics that has been built since the 1950s to deal with describing these types of fluctuating systems, so I was able to leverage that to describe the surface of a nucleus,” Romeo says.
Using spectral analysis, Romeo determined the statistical properties of the wrinkling patterns and the dynamics of wrinkling on the nuclei. This analysis revealed that while the size of the wrinkles changed over time, the wrinkling pattern itself stayed the same.
“To have such consistency emerge from a highly complex process and in such a dynamic cellular environment was surprising,” Romeo says.
After working out how the nuclei wrinkled, the scientists then tested whether they could control the process. Jonathan Jackson, experimental lead on this study and a graduate student at MIT, ran additional experiments on the nuclei to test different theories. The scientists quickly found that by manipulating the cellular environment, they were able to suppress, reverse and even accentuate the wrinkles. Changing the pressure in the wrinkled cells by adding salt increased the wrinkling, while adding water to decrease salt concentration partially reverted the wrinkled nuclei to smooth spheres. Additionally, the scientists found that they could change the wrinkling by inhibiting microtubules, tiny structural elements responsible for generating motion that transports contents within cells and from nurse cells to the oocyte. Reducing this motion (by adding a drug that disrupts microtubules) also reversed wrinkling.
“Changes in the nuclei due to a change in saltiness have been seen before, but I was surprised that, by disrupting microtubule-mediated activity, we were able to reverse the wrinkling,” Jackson says.
In the same way there was a pattern to the wrinkles that appeared during aging, there was a consistency to their pattern when the cells were subjected to different manipulations. The scientists speculate that this consistency in wrinkling might play a role in insulating the nucleus and its precious DNA cargo from the cell’s ever-changing external environment.
“This idea is consistent with the fact that the nuclear envelope is not just a passive DNA container. Instead, its shape plays a direct role in responding to the various stresses that a cell experiences and in turn influences gene regulation,” Imran Alsous says.
While these initial explorations reveal possible clues to how to reverse nuclear wrinkling, any potential medical applications aren’t yet clear. That’s because nuclear wrinkling can be caused by a variety of factors. One example is the genetic disease progeria, which is characterized by premature aging. Nuclear wrinkling is a hallmark characteristic of progeria. However, wrinkling in progeria is partially driven by accumulation of a mutated form of a protein called Lamin A. This is in contrast to the cells observed in this study, where wrinkling is correlated with a decrease in the fruit fly equivalent of Lamin A. Due to experimental difficulties with Lamin A, it remains to be seen whether perturbations to the fruit fly version of Lamin A affect wrinkling.
Indeed, with much still to be learned about the mechanisms of nuclear wrinkling, the research team is keen to continue their work. In addition to continuing to experiment with fruit fly nurse cells, they hope their quantitative approaches could be used by other groups to study nuclear wrinkling in different animals and biological contexts — not only during development, but during disease as well.
“I hope that people will appreciate the insights that physics can bring to understanding complex biological problems,” Romeo says.
“From here, there are a lot of interesting avenues we can continue studying,” Imran Alsous says. “We hope our study — with the approaches we have developed to visualize and analyze nuclear envelope shape — will be useful for shedding light on the connection between genes and geometry.”