The Fruit Fly Photographer
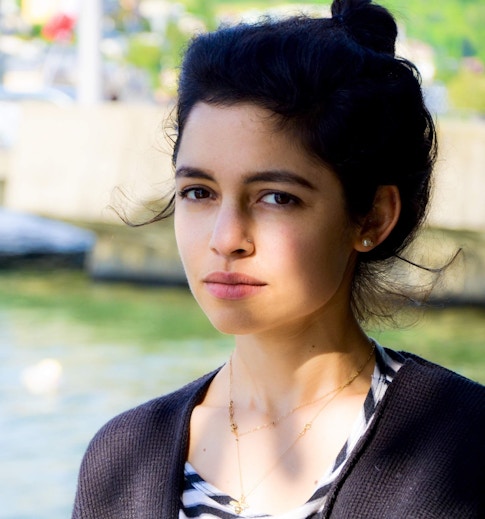
For many people, fruit flies are an annoyance. To Jasmin Imran Alsous, they are a way to make new discoveries.
Imran Alsous is a developmental biologist at the Flatiron Institute’s Center for Computational Biology (CCB). Using cutting-edge technology, she takes high-speed and high-resolution videos of fruit fly eggs and sperm. The resulting images and videos enable Imran Alsous and her collaborators to develop quantitative models to illuminate fundamental aspects of how these cells grow and change with their environment.
Before Imran Alsous joined the CCB’s Developmental Dynamics group, she worked at the Massachusetts Institute of Technology as a postdoctoral researcher in Adam Martin’s lab. She earned her doctorate at Princeton University and her bachelor’s and master’s degrees in chemical engineering at the University of Pennsylvania.
Imran Alsous recently spoke to the Simons Foundation about her work with fruit flies. The conversation has been edited for clarity.
What is the focus of your work as a developmental biologist?
Development is an inherently dynamic process, and that’s true at all levels of biological organization: from genes, through cells, to tissues. I’m part of a group that is interested in characterizing these changes as they unfold during the early development of an organism and understanding what drives them.
Our lab studies an organism’s earliest days — before it becomes an embryo. Successful fertilization and the embryo’s early life are deeply impacted by the quality of the egg, so one of our main topics of interest is understanding how an egg cell is made, how it grows, how it changes shape and the factors that govern this entire process.
To accomplish this, we use a combination of tools: experiments, theory and computing. In my line of work, experiments mainly involve taking lots of pictures and movies of various aspects of early development, typically with a microscope. Watching a process unfold over and over and over again can give you an idea of what’s happening — that idea is what we call a model.
Such a model can then typically be formulated in mathematical terms. Essentially, we use math — often involving a set of mathematical tools called differential equations — to tell the story of what’s happening. It’s the same math you might use if you want to understand where a kicked soccer ball will land. But instead of soccer balls, we’re describing processes such as how the volume or shape of a cell, or the concentrations of proteins in an egg, change over time and how that change affects their activity.
How do you take movies of cells to see how they change?
When I started my studies, microscopy was at a state where we could really start imaging cells and tissue in three dimensions and over time to chart their growth. We primarily rely on confocal microscopes, an optical imaging technique that enables us to take high-quality and colorful images and videos of living samples. Confocal microscopy has been around for over two decades, yet recent advances that leverage artificial intelligence have taken us a step further. We can now acquire much higher-resolution images of eggs or sperm much more quickly, all without disrupting their structure or the processes we want to study.

With confocal microscopy we can now actually see the transport of material sloshing back and forth between connected cells, which happens during the development of an egg. Many of the processes we’re studying were described about 60 years ago, and only now are we able to see them so clearly. It’s a very exciting time to reexamine the fundamental questions people first asked a long time ago.
What fundamental questions are you trying to answer?
During my Ph.D. I was very interested in problems that arise during the formation of the egg. What most people perhaps don’t realize is that eggs are single cells. Even a chicken egg is one cell — a really, really large cell. It’s incredibly hard to make a cell that size — and not something that can be done alone. To become that large, the egg grows while remaining physically connected to other cells, which are called sister cells or nurse cells. I examined how these cells work with eggs to help them grow so large. We studied this using fruit fly eggs, which are a commonly used animal because they’re easy to work with and well understood genetically.
Recently, I switched my area of focus from the fruit fly egg to their sperm. The fruit fly is a little animal, but its sperm are comparatively massive. While the fruit fly itself is only about 3 millimeters long, the tails of its sperm stretch to 2 millimeters in length. Their length poses new challenges for understanding how these cells propel themselves — both inside the male and in the female. One of the questions we want to answer is: How does such a large cell swim?
To understand this, we started taking movies of fruit fly sperm. We were amazed to see how the sperm — not one sperm but hundreds of them — all swim past each other and never entangle in a sort of complex choreography. In male fruit flies, sperm are largely confined to a kidney-shaped pouch before they’re released into the female. But once deposited in the female, they swim into one of two female storage organs, one of which looks like a portobello mushroom. Storing sperm in the female for considerable amounts of time is not unusual in the animal kingdom. In flies, sperm storage can facilitate competition between sperm from different males.

To understand how sperm swim harmoniously in these different locations, we’re taking videos of all these ways fruit fly sperm move in differently shaped environments and making models to describe how they swim.
I’m doing all of this in very close collaboration with people who study fluid dynamics and biophysical modeling, including Brato Chakrabarti, a fellow Flatiron researcher who works in CCB’s Biophysical Modeling group under Michael Shelley’s guidance. We’re working together to translate what we see in these videos into a quantitative picture. We’re in the early stages at this point, but eventually our goal is to develop a model that not only captures what we see in the movies, but also makes predictions and guides future experiments.
Are there connections between your work and the study of fertility and fertility issues?
It’s not hard to see there could be connections. In fact, research on fruit fly egg and sperm development has informed our understanding of similar processes in mammals, including humans, and hence their fertility. There are lots of differences as well across species, so one must tread carefully. We nonetheless hope that the quantitative and systematic approaches and techniques we’re establishing here could be applied to better understand and hopefully alleviate challenges with fertility.