
A New Era in Neural Recording
During the pandemic, graduate student Anna Lebedeva had the eerie experience of working in the lab entirely alone. The University College London campus was shut down, but Lebedeva received special permission to continue a unique set of experiments. Prior to the pandemic, she had implanted mice with Neuropixels 2.0 probes, the latest iteration of a high-density silicon electrode capable of recording from hundreds of neurons simultaneously. Her lab was one of only a handful around the world that were testing the new probes, so every animal housed a potential treasure trove of data.
As her mice viewed slideshows of images, Lebedeva watched bursts of activity unfold in the visual cortex in real time. “I recorded some of these precious mice for more than 300 days, and spent the rest of the pandemic analyzing the data,” says Lebedeva, who works in Matteo Carandini’s lab. Lebedeva, Carandini and a consortium of scientists from different labs published the first round of Neuropixels 2.0 results in Science in April.
They showed that the electrodes could reliably record from the same neurons for months, a capability they hope will open new vistas for researchers studying learning and memory. Lebedeva, for example, is using the technology to analyze how neurons’ activity shifts over time as an animal learns. “This is the kind of fundamental question that had been impossible to answer because it was too ambitious,” says Carandini, an investigator with the Simons Collaboration on the Global Brain. “Up until now, we hadn’t been able to do a one-to-one before and after comparison.”
Since the first iteration of the Neuropixels technology, Neuropixels 1.0, became commercially available in 2018, thousands of probes have been shipped to more than 450 labs around the world. The ability to record directly from many cells at different sites in the brain allows scientists to look at the brain in fundamentally new ways — they can test previously untestable models for how cells encode spatial information, for example. Recording from multiple probes in different parts of the brain simultaneously has revealed that neural responses once thought to be specific to certain areas, such as the motor or visual cortex, can be found all over the brain, hinting at a much more global picture of brain function.
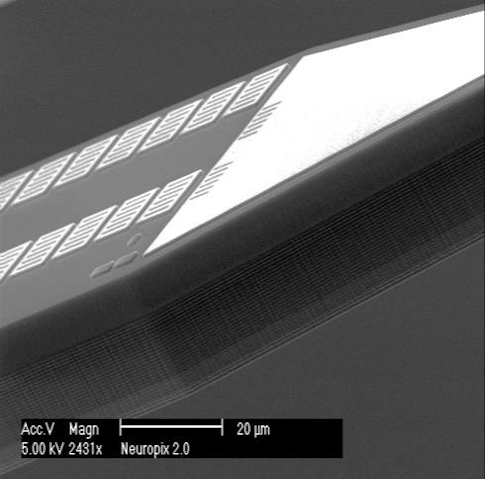
“To study one brain region at a time is like the proverbial blind men with the elephant, where no one can understand what the elephant is by examining only a small part,” says Nick Steinmetz, a neuroscientist at the University of Washington and one of the researchers on the Science study. Neuropixels and other high-density electrodes are bringing the whole elephant into view — at the resolution of every wrinkle.
Given the relative novelty of the technology, Steinmetz predicts that the most exciting findings are likely yet to come. “I hope that within a year or two, we may see that Neuropixels recordings lead to an emerging understanding of the types of computations carried out by large populations of neurons.”
Of course, with new power come new challenges. It’s impossible to manually analyze long-term recordings of hundreds to thousands of neurons, so scientists are developing new methods to process and analyze recordings from large populations. But they are also struggling to figure out how to best assess the accuracy of those methods.
A new kind of probe
One of the biggest challenges in designing high-density recording probes is wiring. Each sensor electrode is attached to its own wire, which ferries electrical signals to a chip that amplifies and digitizes the information. The recording capacity of typical silicon probes has traditionally been limited by the number of wires that could be attached to the probe. Neuropixels cleverly removed this barrier by integrating the sensors and the digitizing chip into one silicon device. “Neuropixels freed us from the tyranny of cables,” says Carandini.
This innovation was made possible by working with commercial-grade chip makers. Both commercial computer chips and high-density silicon electrodes are made using the same technology — microelectromechanical systems (MEMS). But large commercial foundries have traditionally had little interest in small volume projects for neuroscientists, and Neuropixels predecessors were manufactured using older technology in university MEMS foundries.
In 2013, Tim Harris, director of applied physics at Janelia Research Campus, in Ashburn, Virginia, convinced four nonprofit agencies — Janelia, the Allen Institute, the Gatsby Foundation and the Wellcome Trust through a grant to the University College London — to donate $5.5 million in grants to fund the design and manufacture of a new type of probe. Harris enlisted a commercial-quality foundry, called Imec, sponsored by the Flemish government, allowing for a more efficient and robust design. “That was a sea change,” says Mattias Karlsson, cofounder of SpikeGadgets, a company developing electronics that pairs with new probes. “Everything is in one monolithic silicon device.”
The Neuropixels 1.0 probe costs about $1400 and packs nearly 1,000 recording sites onto a single shank 10 millimeters long and a cross section of roughly 1,400 microns square, which spans the full depth of a rat brain. Roughly a third of the sites can be used at any given time, due to limitations of wiring and electronics. But scientists can record from different parts of the brain without physically moving the probe by changing which sites they use.
Over the last two years, researchers have used Neuropixels probes to explore previously impractical or inaccessible questions. During a talk at the virtual Neuronal Ensembles symposium in May, Edvard Moser, a neuroscientist at the Norwegian University of Science and Technology (NTNU) in Trondheim, outlined how Neuropixels has finally enabled their lab to test a model of spatial coding known as the attractor network theory. Theorists had posited that continuous attractor networks underlie spatial tuning in grid cells, as ring attractors do for head direction cells. This theory predicts that the neural activity of groups of grid cells would form a torus when plotted in high dimensional space. But testing the theory requires researchers to record from many cells. With Neuropixels, Moser’s lab recorded from 149 cells from a single grid module in the medial entorhinal cortex during foraging and other tasks and found that neural activity from a single module indeed spans a torus. The toroid population map persists in the absence of sensory inputs, such as during sleep, and when animals are navigating spaces with complex topology.
Other researchers have used multiple probes to explore how information is encoded across the brain, revealing that movement-related activity can be found not just in the motor and somatosensory areas but across the brain, suggesting that an animal’s ongoing behavior influences how it processes information and makes decisions.
When Neuropixels was first developed, Harris worried about their decision not to license the technology to a commercial vendor. That in essence means the probes are cheaper to buy, but Imec, the manufacturer, does not provide the user support typical of most commercial devices. “We knew the community would have to deal with it at some level,” Harris said. “I was afraid it might collapse, but we’re doing fine.” For support, users turn to the Neuropixels Slack channel, which has about 750 members, Harris says, or reach out directly to other users or to his group.
Taking the long view
Harris knew from the get-go that his team would ultimately develop a smaller and denser device. In 2019, the first batch of Neuropixels 2.0 probes was delivered to the labs that helped develop it. Lighter and roughly one-third the size of their predecessors, and with four shanks instead of one, the new probes are more practical for long-term use in freely moving mice. Two probes can be attached to a single head stage, generating a device with more than 10,000 sensors — 768 of which can be used simultaneously — weighing just over 1 gram. Researchers can adjust what part of the brain they’re listening to by changing the recording sites, and they can use multiple probes in the same animal.
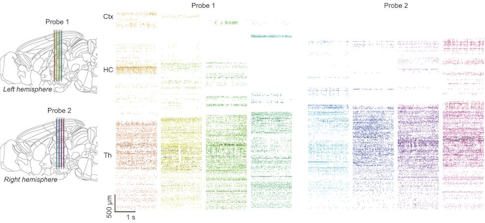
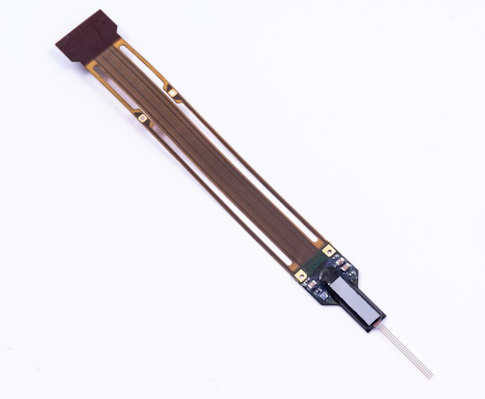
“It’s a tremendous step forward for studying local population coding within a brain region,” Steinmetz says. “It allows a much denser sampling of local populations — every advance in population size enables us to see more things.” The consortium of organizations that developed the new probe includes Janelia, the Wellcome Trust (through a grant to UCL), Neuro-Electronics Research Flanders in Belgium, the Kavli Institute at NTNU, and the Champalimaud Center in Portugal — principal investigators from those institutions got first crack at the device.
A major challenge for long-term neural recordings with stiff electrodes is drift — the brain can move relative to the probe, which is affixed to the skull, making it difficult to track the same neuron over time. The Neuropixels 2.0 developers tackled this problem with both hardware and software. Recording sites are stacked closer together and in a column, rather than in the checkerboard arrangement of Neuropixels 1.0. As brain tissue moves up and down, different sensors along the vertical probe can continue to record from the same neuron. Specialized software designed to pick out the characteristic electrical traces of individual cells enables scientists to record from the same neuron for weeks.
To confirm that they were indeed recording from the same cells day after day, Carandini, Steinmetz and collaborators checked the cells’ neural signatures — their unique response to specific images. They found they could record from 90 percent of neurons stably for two weeks and 80 percent stably for two months. “We think we have largely solved the stability problem, depending on the condition of the recording,” Steinmetz says. Though researchers had previously been able to look indirectly at activity in the same neuron for weeks or months using optical recording technologies, those techniques have lower temporal resolution and are largely limited to head-fixed animals. Neuropixels 2.0 can be used in freely moving mice.
The ability to stably record from hundreds of individual neurons over months makes it possible to study fundamental properties of the brain, such as why neural activity varies under seemingly identical conditions. Steinmetz’s lab is examining why individual neurons respond differently to the same stimuli over time. “We hypothesize that some variability might be accounted for by internal states,” he says. “We are recording lots of neurons over different parts of the brain and asking if neurons in different parts of the brain account for some of the variability in response.”
Despite these advances, long-term recording capacity can be hit or miss. For reasons researchers don’t completely understand, perhaps because of damage to the brain, “we see a slow decay in the number of neurons recorded over time,” Carandini says. The part of the brain and type of cell being targeted may play a role. Some researchers say it’s too soon to know how broadly the long-term recording software will work. Loren Frank, an SCGB investigator at the University of California, San Francisco, who is developing an alternative type of high-density electrode, acknowledges that Neuropixels are a huge advance, particularly for acute recordings. But he is concerned they won’t work well under some circumstances, such as when neurons go silent for a while and then come back on. “I don’t know if the algorithm can deal with that case well,” he says.
Imec is now optimizing manufacturing for the 2.0 probes, producing 1000 ‘engineering prototypes.’ Harris estimates probes will be available for order in the second quarter of 2022 for roughly the same price as Neuropixels 1.0.
Neuropixels’ new frontiers
As soon as primate researchers got wind of Neuropixels, they clamored for a version of their own. HHMI obliged, funding development of a larger probe sized for primates, which proved to be an unusual challenge. The most advanced integrated circuits, like those used for video game processors, are about an inch square. But primate probes require a shank nearly 2 inches long, so developers had the tricky task of aligning four 1-inch-square chips, Harris says. Recording shanks are also much thinner than integrated circuits, generating stress on the material.
A prototype of the probe, dubbed Neuropixels NHP, is now being tested in a handful of labs. So far, scientists are singing its praises. “It’s a game changer,” says Michael Shadlen, an HHMI investigator and a neuroscientist at Columbia University’s Zuckerman Institute. “For the first time in primates, we can see in one trial the kinds of signals we used to infer by averaging over many trials.” In research presented at the Cosyne meeting in March, Shadlen’s group examined how neurons accumulate evidence during decision-making.
“We have found they work spectacularly well with relatively little fuss,” says Mark Churchland, an SCGB investigator at the Zuckerman Institute who shares a postdoc with Shadlen. “The ability to get that number of neurons simultaneously is for many purposes transformative.” He expects to use Neuropixels for the vast majority of his experiments when they are broadly available. “The ability to iterate experimental design with recordings is incredibly promoting of creativity,” he says.
Neuropixels NHP will be especially useful for studying internal cognitive processes, such as decision-making, where it’s difficult to know exactly when an animal made its decision. “Averaging neural activity across trials can distort or disguise key events because the moment when you commit to a decision might be different on different trials,” says Churchland. Neuroscientists have developed various computational methods for inferring information from single trials, but Neuropixels allows them to look at it directly. Preliminary research from Churchland’s lab, posted on the bioRxiv in May, suggests that the motor cortex has a surprisingly high number of degrees of freedom.
Harris anticipates that Neuropixels NHP will be produced commercially, but he doesn’t yet know when or how much they will cost.
Harris and Steinmetz are also exploring new sensor architectures, trying to find the optimal sensor spacing for recording information. “We want to understand how big and how far apart sensors are in the perfect probe,” says Harris. That effort, called Neuropixels Ultra High Density, is funded through Allen, Janelia and UCL. It is intended as a research tool, not a product, Harris says. The National Institutes of Health is sponsoring a follow-up project to make probes in different sizes and to determine if the technology can identify neuron type using the pattern of electrical signals detected in an array of very dense small sites.
The final device on the Neuropixels frontier is Neuropixels NXT, which Harris calls “the last generation of probes worth making.” Neuropixels 2.0 has 5,000 sensors, but scientists can only listen to 384 of them at a time, a limit imposed by the size of the electronics that can fit on an animal’s head. “Why not fix it to listen to all of them?” Harris says. With a $13.5 million grant from the BRAIN Initiative, Harris and collaborators will design a new probe from scratch, using the most advanced microfabrication techniques available. It will be about the same size as Neuropixels 2.0 but have the capacity to use four times as many channels at once — roughly 2,000 per probe — and be small enough to use multiple probes in a freely moving mouse. Harris expects prototypes to be available in 2024, with commercial versions to follow in 2025.
A New Era in Neural Recording Part 2: A Flexible Solution
Flexible electrodes offer an alternative approach to high-density recordings. Both methods face challenges in how to best process data.