
In Senescent Cells, a Promising Route to Slowing Brain Aging
In the early 2000s, Darren Baker was studying cancer by working with mouse models whose chromosomes failed to segregate properly, a risk factor for tumor formation. One of the strains he created had a curious phenotype — premature aging. None of the typical drivers of premature aging known at the time, such as an inability to repair DNA damage, short telomeres, or issues with mitochondria, offered a satisfactory explanation. “Our model never fit that,” says Baker, a biologist at the Mayo Clinic in Rochester, Minnesota. Instead, they found that the animals had accumulated a lot of so-called senescent cells, cells that have stopped dividing.
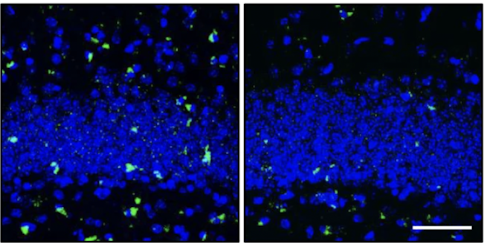
In the years since, Baker and others have uncovered growing evidence that senescent cells play an important role in aging, both broadly and in the brain. A number of studies have shown that these cells accumulate with age and in different neurodegenerative diseases. They release inflammatory factors, which can harm neighboring cells. And most intriguingly, eliminating them in mice either genetically or with drugs can delay symptoms of different diseases and may improve cognitive function. “Senescence is increasingly recognized as a fundamental mechanism of aging and contributes to age-related disease,” says Yousin Suh, a biologist at Columbia University and an investigator with the Simons Collaboration on Plasticity and the Aging Brain.
But studying senescent cells presents a serious challenge. They are rare and notoriously difficult to identify, which has hampered efforts to answer basic questions about their role in aging. “In mice, we don’t have great markers of senescent cells or ways to characterize how they change over time,” Baker says. “We don’t know how long senescent cells live in tissue, whether there are milder and more extreme forms of senescence, or why they even come around in the first place.”
To address those questions, researchers are now developing new technologies for profiling and targeting senescent cells, which will help them track more precisely how the cells change with age, disease and, perhaps most importantly, treatment. Ultimately, researchers hope a better understanding of senescent cells in the brain will aid development of new drugs that can kill or slow senescent cells, some of which are already in clinical trials for neurodegenerative disease. “It’s a really exciting time in our field for determining how senescent cells modify the brain in the context of aging and disease,” says Marissa Schafer, a biologist at the Mayo Clinic. “New platforms will help identify the location and identity of senescent cells and will be really useful for answering some of these questions.”
Hayflick’s limit
The biologist Leonard Hayflick first described the concept of senescence in the 1960s, showing that fibroblasts growing in culture divided a fixed number of times and then stopped. Hayflick proposed at the time that senescent cells play a role in aging, but it’s only in the last 20 years that researchers have had the tools to study senescent cells in the body. We now know that senescence strikes different cell types and can be triggered by different factors, including DNA damage, telomere damage and oxidative stress.
The impact of senescent cells runs the gamut from helpful to harmful. The cells aid in wound healing, for example, sending inflammatory factors to the site of a wound. They play an important role in development, secreting factors involved in neural tube development. Senescence can also act as a tumor-suppressing mechanism, arresting division in cells with DNA damage.
But in the long term, and particularly in aging and age-related diseases, senescence can be detrimental. Senescent cells are problematic both because they no longer replenish tissue with new daughter cells, and because they produce inflammatory cytokines and other molecules, a property known as the senescence-associated secretory phenotype or SASP. Secreted factors can harm neighboring cells, triggering cell cycle arrest, disrupting stem cells and impairing regeneration. “When you’re young, it’s good to have this anticancer mechanism, but when you’re old, it does all those terrible things,” Suh says.
Under normal circumstances, the immune system clears senescent cells. But for reasons scientists don’t yet fully understand, the cells tend to accumulate with age. “Maybe we simply generate too much or the ways to remove senescent cells become less efficient with age,” Suh says. The accumulation of senescent cells may then create a feedback loop, as they secrete inflammatory cytokines and potentially generate more senescent cells.
One of the biggest challenges in studying senescent cells, particularly in the brain, is simply identifying them. “I think the brain is one of the organs we know relatively the least about senescent cells,” says Valery Krizhanovsky, a biologist at the Weizmann Institute of Science in Rehovot, Israel. Senescent cells are rare, even with age and disease, and what senescence looks like varies from one cell type to another. Moreover, different methods of triggering senescence, such as oxidative stress, DNA damage or epigenetic changes, can induce different senescence phenotypes even in the same type of cell, Suh says. “No single marker can identify senescent cells in vivo; it really depends on the cellular context.” Researchers often rely on markers tied to the cell cycle, such as P16 and P21, proteins which at high levels can block cell division.
Some of the clearest evidence of senescent cells’ role in aging comes from efforts to eradicate them. About a decade ago, Baker and collaborators at Mayo engineered a genetic construct, dubbed INK-ATTAC, that kills senescent cells expressing P16 when the animals are fed a certain drug. Removing these cells slowed age-related decline in the heart, kidneys and other tissues, extended life span in normally aging mice, and slowed disease progression in a rapidly aging mouse model. Though scientists are still examining exactly how removing senescent cells boosts tissue health, slowing the release of harmful senescence-associated factors may be a key component. “Tamping down inflammation, either by eliminating the cells or the secretion of proteins, can have a beneficial effect,” Baker says.
Senescence in the brain
In their early INK-ATTAC studies, Baker’s team didn’t extensively test brain function. But they did see subtle cognitive differences. “One thing that really changes when you remove senescent cells in mice, they run around more,” Baker says, though researchers don’t yet know why. It could be better muscle function, or it could stem from changes in the brain.
To date, most studies examining the role of senescent cells in the aging brain have focused on mouse models of neurodegenerative disease. Baker’s group, for example, has found that an Alzheimer’s model that develops aggressive neurodegenerative disease in the first year of life accumulates senescent glia. Halting development of senescent cells before the animals show signs of neurodegeneration, the equivalent of treating someone in their 20s, “profoundly delays degeneration, suggesting the senescent cells promote dysfunction,” Baker says. The researchers are now examining whether targeting senescent cells after degeneration has already begun can still slow decline. “When can we intervene? How late is too late?” Baker asks.
Using a different Alzheimer’s mouse model, Miranda Orr, a neurobiologist at Wake Forest University School of Medicine in North Carolina, and collaborators also showed that targeting senescent cells reduces tau pathology. Though the two studies identified different types of senescent cells, both showed that clearing senescent cells reduces signs of pathology, which is exciting, Schafer says.

Researchers say it’s too early to know how applicable those findings are to normal brain aging — senescence in mouse models of neurodegenerative disease and accelerated aging may be very different from what happens in the absence of disease. However, new research does point to a role in brain aging. In a study published in Aging Cell in 2021, Diana Jurk, a biologist at Mayo, and collaborators showed that removing senescent cells can indeed improve brain function in old mice. Single-cell and single-nucleus RNA sequencing in these animals revealed that P16-positive senescent microglia, oligodendrocytes and oligodendrocyte precursors accumulated in the hippocampus with age. Treating old mice to remove these cells — both genetically and with a drug cocktail — reduced P16 and the release of inflammatory factors in microglia and improved performance on a spatial memory test. “Our data provide proof of concept that senescent intervention can be a therapeutic avenue for alleviating age-associated cognitive impairment,” Jurk says.
Researchers are now trying to understand which cell types and brain regions are the biggest drivers of brain decline. Scientists initially thought that neurons — terminally differentiated cells that no longer divide — could not become senescent. But that notion began to change in 2012, when Jurk and collaborators showed that post-mitotic neurons in the mouse brain displayed features similar to those of senescent cells. Researchers have since demonstrated that a number of non-dividing cells can become senescent, including hepatocytes and cardiomyocytes.
Microglia also show signs of senescence, and both Schafer and Jurk suspect they play a central role. “They are a major mediator of pro-inflammatory dysfunction in brain aging, but how they disrupt circuit function has not been shown yet,” Schafer says. Identifying senescent microglia is especially challenging, because microglia become inflammatory under stress, making it difficult to distinguish stressed microglia from senescent ones. Baker’s team is using single-cell RNA sequencing to characterize individual cells and find markers that are unique to senescent versus activated microglia.
It’s also unclear which of the various types of brain cells become senescent first. Some people think neurons are the initiators, others that myelin precursors become senescent first, triggering myelin loss and neural dysfunction, says Baker. “We are all coming to different answers,” he says. “But the commonality looks like the senescent state can be influenced positively by removing the cells.”
Baker, Jurk and Schafer hope that new tools under development to more precisely identify and clear senescent cells will help answer some of these questions. For example, both INK-ATTAC mice and senolytic drug treatment target P16-positive cells all over the body, so scientists don’t know whether the cognitive benefits derive from removing senescent cells from the brain specifically. The researchers are now breeding mice in which they can selectively clear P16-positive microglia, oligodendrocytes or astrocytes to examine the impact of targeting each cell type. Schafer, Orr and others are developing new methods for analyzing multidimensional markers of senescence in intact tissue rather than in dissociated cells, which will allow researchers to more effectively assess which cell types are affected and how senescent cells influence their neighbors.
Baker’s team is developing methods to drive specific cells to senescence. “If I promote senescence in astrocytes, does it cause issues in the brain?” Baker says. “That’s what we’re doing right now.” He says that inducing microglia to become senescent has proved challenging. “We can make them inflammatory, but if we try to put them into an arrested state, they go more into a state of cell death,” he says.
Senolytics and senomorphics
Translating senescence research into novel therapies will require drugs that can specifically target senescent cells; these are known as senolytics. A related class known as senomorphic drugs lowers harmful secretions to reduce the spread and impact of senescent cells.
James Kirkland, a physician and biologist at Mayo, began searching for senolytic drugs almost two decades ago. His team has identified 40 or so compounds that target parts of a survival network that stops some senescent cells from committing suicide. To expedite clinical testing, the researchers have focused their efforts on approved drugs or natural products with existing safety data, such as the combination of Dasatinib, a chemotherapy drug, and Quercetin, a flavanol found in plants. (Kirkland and collaborators have shown that Dasatinib and Quercetin can increase klotho, a longevity factor, in mice and humans.) Kirkland is part of a clinical trial network running a number of trials for senolytics, including some for Alzheimer’s disease. One early-stage clinical trial at the University of Texas Health Science Center at San Antonio is analyzing how much of that combination senolytic gets into the brain and how it affects senescent cells and other markers in people with Alzheimer’s disease.
Researchers hope that more basic research on senolytics will help guide clinical efforts. For example, it’s not yet clear whether removing certain senescent cells could be harmful. When Baker first started studying senescence, he worried that killing cells in a model already subject to neurodegeneration would further harm the brain. “But we found that the cells we are killing have a negative influence, and getting rid of them helps other cells.” He notes that short-term treatments to remove senescent cells may be adequate for reducing their impact, balancing the helpful role of senescent cells with a short-term cleanup to prevent problems. Treatments could be stopped before surgery, for example, when wound-healing capacity is important.
Some researchers are unsure about targeting neurons in particular. Senescent neurons are still part of the neural network and seem to participate in neural coding, Jurk says, though they don’t function as well as their non-senescent counterparts. “If we kill 3 to 4 percent of neurons, would we interfere with certain things?” Jurk says. “Would someone take a drug and forget stuff because we eliminated neurons?” She suspects the best option might be clearing senescent microglia, which would improve the overall environment for neurons.
Indeed, some evidence suggests that senescent cells can be helpful, Krizhanovsky says. A study published in Nature Neuroscience in 2020 found that buildup of natural killer cells, which eliminate senescent neuroblasts, worsened cognitive function, while depleting the natural killer cells improved brain function. “To maximize the benefit, we need to understand how each cell type contributes and how to target cell types that are detrimental,” he says.
Another important question is whether specific senolytic drugs cross the blood-brain barrier — and whether they need to. Scientists don’t yet know whether the cognitive benefits of removing senescent cells stems from targeting the brain or the periphery, and research is ongoing as to whether the aging process itself may impact the strength of this barrier. (Peripheral factors can have a profound effect on the brain. For more, see “Exploring How Young Blood Helps Reverse Signs of Aging in Old Brains.”)
Answering these questions will require more effective methods for tracking human senescent cells. The National Institutes of Health aims to fill that gap with the Cellular Senescence Network (SenNet), a new effort to map senescent cells in human tissue across the life span. In one SenNet project, Suh and collaborators will analyze brain tissue from a sudden-death cohort ranging in age from 20s to late 80s, integrating diverse single-cell data from different cell types, including neurons, microglia, astrocytes and oligodendrocytes. “We will see what happens during normal aging in the brain — which are the senescent cells, what are the molecular and cellular characteristics,” Suh says. So far, they have found that cells expressing p21, a senescence marker, increase with age in multiple cell types in the brain, including astrocytes. Intriguingly, one young person who died of opioid toxicity had much higher levels than old people.
To develop more accurate biomarkers for senescence, they are analyzing whether cells with existing markers, such as P16, P21 and SASP, tend to express other markers as well. Researchers will evaluate candidate markers using induced pluripotent stem cells, manipulating senescence markers to see if they can recapitulate senescent phenotypes. They’ll validate their markers using senolytics, assessing whether treatment with these drugs eliminates proposed markers of senescence. The ultimate goal is to develop biomarkers specific to each cell type and location in the brain. In addition, they will assess whether neighboring cells also become senescent or show other harmful phenotypes.
Orr is working on similar efforts to develop combination biomarkers. In a study published in Nature Aging in December, Orr and collaborators used gene patterns known to be important in other types of senescent cells to search for cells in postmortem human brains showing these same patterns. The vast majority of senescent cells they found were excitatory neurons, and many also had neurofibrillary tangles, which often occur with dementia. The researchers are now using machine learning approaches to identify new combinations of markers that can identify diverse types of senescent cells.
Researchers hope these tools will ultimately aid drug development and testing. “To know the drug worked, you want to have good biomarkers,” Suh says. “That’s what we will provide for the brain.”