How Electrons and Nuclei Work Together to Build Our World
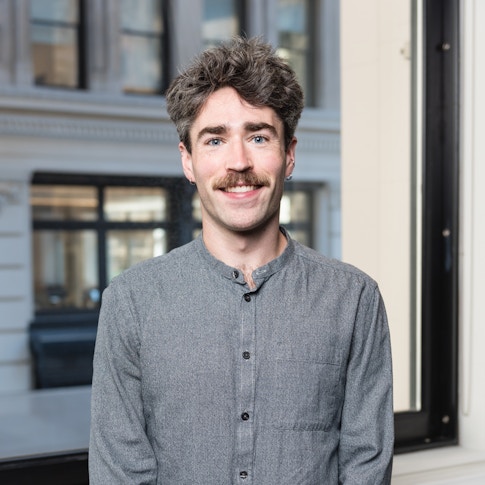
When he was in high school in Connecticut, Eric Arsenault was fortunate to have amazing chemistry and physics teachers who were passionate about their subjects. While the two fields are usually taught as separate disciplines, in reality, chemical and physical processes work together to fuel much of what we see in our world. Now a first-year Simons Junior Fellow, Arsenault is a physical chemist whose work straddles the two fields.
Arsenault earned a doctorate in physical chemistry from the University of California, Berkeley, and holds undergraduate degrees in both physics and chemistry from Wesleyan University. He is now a postdoctoral fellow at Columbia University in the lab of Xiaoyang Zhu. In addition to his current Simons Society of Fellows Junior Fellowship, Arsenault also received support from the National Science Foundation while at Berkeley.
Arsenault and I recently discussed his work, and our conversation has been edited for clarity.
What are your core scientific interests?
At the most basic level, I want to understand how things work. In my case, this means I am interested in quantum mechanics and dynamics. I find what happens in the physical world — particularly at microscopic scales — to be most fascinating. I do this work through the lens of physical chemistry, which is the study of the physical phenomena that underlie how matter behaves. As a physical chemist, I get to design experiments that help bring us closer to understanding the physical underpinnings of chemical processes, such as how leaves absorb light during photosynthesis.
I understand that photosynthesis was a focus of your doctoral work at Berkeley. Can you describe that work?
We all remember learning about photosynthesis, the process by which leaves absorb light in order to convert water and carbon dioxide into sugar. This is arguably one of the most important chemical processes on the planet. But we still don’t fully understand the complex initial cascade of events that are triggered as photons (particles of light) from the sun are absorbed by pigment protein complexes in a leaf.
At Berkeley, I delved into a puzzling aspect of photosynthesis. Energy captured from the sun must travel from one pigment-protein complex to another in order to make it to what is called a “reaction center”: the place where the conversion of light energy to chemical energy begins. The ability of photosynthetic organisms to control energy in this way has never been fully elucidated, so I used a technique generally known as ultrafast spectroscopy — which employs pulsed lasers to study the dynamics of molecular or material systems — to investigate.
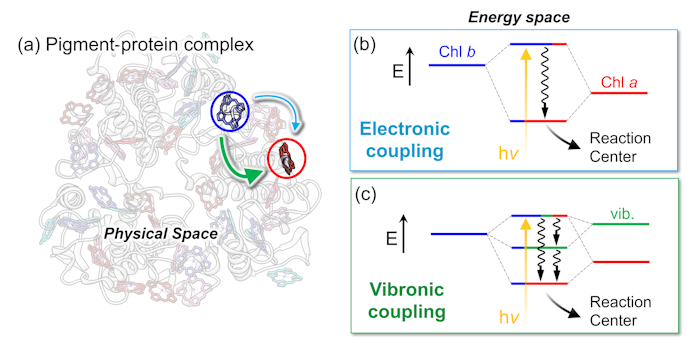
The chemical processes of photosynthesis happen extremely quickly, sometimes on the order of femtoseconds — quadrillionths of a second. But ultrafast spectroscopy enabled us to measure these fast-moving events. We could shine a burst of laser light on a collection of proteins extracted from a leaf and then see what happens as the absorbed light energy is dispersed throughout the protein complex. We discovered that this energy flow requires cooperation between nuclei and electrons to efficiently transport the energy to where it can be properly utilized.
What are you working on now?
My current focus at Columbia may at first seem like a radical departure from my doctoral work on photosynthesis. In fact, it is a continuation of my quest to understand how electrons and nuclei work together to dictate the flow of energy or charge.
These days I am studying atomically thin semiconductors that, when overlaid on top of each other, form what is known as a “moiré pattern.” In physics, a moiré pattern is a general phenomenon that arises when near-identical patterns are overlaid onto each other. This then leads to the creation of a larger interference pattern. Recently, researchers have demonstrated that semiconductor systems endowed with such a property can trap, or localize, electrons. This is fascinating, as typically we understand electrons as quantum mechanical objects that are highly delocalized. I want to understand the interactions that give rise to this electron localization.
From a theoretical standpoint, findings from this work could have implications for quantum computing or other technologies, though applications are many years away, as our understanding of these systems is still in early development. A dream of mine would be to leverage these sorts of systems to potentially improve device efficiencies.
Are there other potential applications related to your research?
As I was studying the physical and chemical processes of photosynthesis at Berkeley, I was also working on how to rethink solar energy sources. Developing a bio-inspired design aimed at improving the efficiency with which we can harvest sunlight would be the ultimate goal. Globally, energy demand is increasing exponentially, so this is an urgent problem. At a base level, current solar cells are built with semiconductors, so it seemed like a good idea for me to understand their properties more fundamentally. I was struck by the crystalline, ordered structure of semiconductors and how they could not be more different from the wet, noisy environment of biological systems. How do we reconcile this difference — and use that knowledge to build improved sources of energy? This is one question that has since led me to my current research at Columbia.
Additionally, we know that a growing fraction of this increasing energy demand is coming from our technologies. If we could improve device efficiencies, perhaps we could reduce energy demand. All that said, whatever applications come from this, my own personal interest is rooted in the sheer satisfaction of better understanding how things work.
Finally, what are your thoughts about the Simons Junior Fellowship?
I have many thoughts, all of them good.
First, it’s been extremely rewarding to speak to both Senior and Junior Fellows, who are each experts in their respective fields. In speaking with Junior Fellows, I can get practical advice about life as a postdoc, as well as more forward-thinking advice about how to run my own lab someday. It’s also been wonderful to learn about scientific disciplines other than my own. My conversations with other physical chemists inevitably devolve into esoteric discussions, but that can’t happen when I am describing my work to someone who studies neuroscience, molecular biology or mathematics. It forces me to hone my skills at explaining the purpose and goals of my research in ways that anyone can understand and get excited about.
Relatedly, the weekly Simons Foundation Presidential Lecture series and accompanying dinner, which all Fellows attend, is a wonderful opportunity to be exposed to the beauty of science, and it has been foundational in fostering our community of scholars. And of course, the unrestricted support that the Simons Foundation offers allows me to pursue bold questions — to follow the science wherever it leads. And for that, I am truly grateful.