The Radiant Research of a Black Hole and Massive-Star Modeler
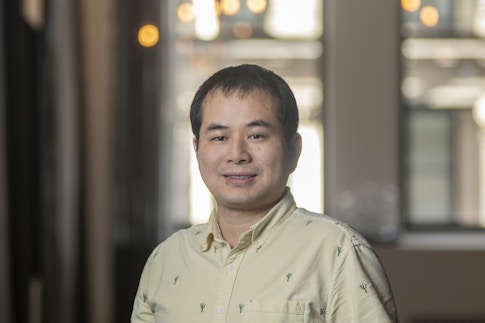
Black holes often conjure up images of dark voids in space. But for theoretical astrophysicist Yan-Fei Jiang, they’re anything but. Dressed in layers of blue, red and yellow, his simulations of black holes are full of color.
At the Flatiron Institute, Jiang develops complex three-dimensional computer simulations to study the disks of material that swirl around black holes and to model other extreme cosmic environments in which light fights against the crushing force of gravity. This work is helping Jiang and his collaborators understand some of the most extreme environments in the universe.
Jiang has been an associate research scientist at the Flatiron Institute’s Center for Computational Astrophysics (CCA) since 2019. Prior to joining the CCA, he was a KITP fellow at the Kavli Institute for Theoretical Physics at the University of California, Santa Barbara. Jiang earned his doctorate in astrophysics from Princeton University and his bachelor’s in physics from Tsinghua University in Beijing.
Jiang recently spoke to the Simons Foundation about his work simulating the cosmos. The conversation has been edited for clarity.
What projects are you currently working on?
My main focus is developing general-purpose computer simulation tools to solve equations of radiation and magnetohydrodynamics in all different kinds of astrophysical systems. Radiation equations are those dealing with the fundamental physical process of energy transfer. In the astrophysical setting, these equations are used to describe how a hot gas emits photons and how that energy is transferred outward. These equations also describe how photons get absorbed or scattered by the gas, which gives the gas momentum. Magnetohydrodynamics, on the other hand, is the study of the magnetic fields of fluids that conduct electricity. The fluids I deal with are gases and plasmas, which is a fourth state of ionized matter.
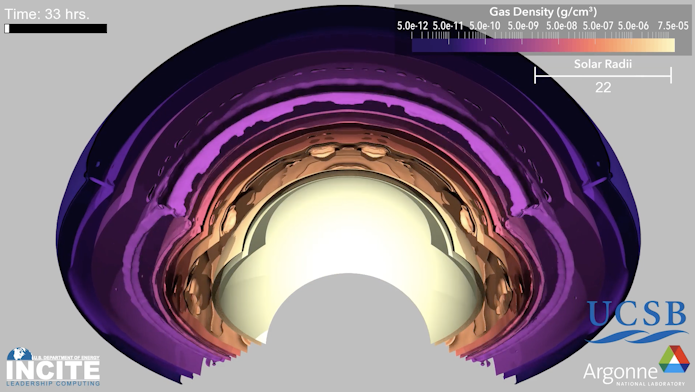
By clicking to watch this video, you agree to our privacy policy.
Right now, I’m focused on creating 3D simulations that include radiation and magnetohydrodynamic components to study two different kinds of astrophysical systems — stars and compact objects, which include black holes and neutron stars. For black holes I’m modeling their accretion disks, which are flattened disks of material that coalesce around a black hole, pulled in by its gravity.
Black holes play an important role in the structure and evolution of galaxies. They are also important sources of gravitational waves. However, accretion disks around black holes are hard to model theoretically — no single model can completely explain how all black holes grow. The focus of my work is to improve simulations of the accretion process. In my simulations, I include radiation equations to describe how material around a black hole heats up, emits light and interacts with the magnetic fields. The big idea is my simulations can eventually be compared with observations directly to see if we’re correctly understanding the mechanisms at play around a black hole.
I also work with 3D simulations to understand the surface structure of massive stars, which can be 20 to 100 times more massive than the sun at different evolutionary stages. At these masses, a star’s surface layer is supported by radiation pressure, which means the pressure due to the scattering and absorption of photons is even larger than the force of gravity. This makes the surface layers very unstable. With 3D simulations, we can study how this layer will behave under changing conditions, such as fluctuations in the luminosity or temperature. This is critical for us to understand how these stars look and how they will evolve. Our knowledge of 3D structures of stars can also be incorporated in the traditional 1D stellar evolution models.
How do you make these simulations?
We start with a 1D model. We use different 1D models that have been developed and made public by the scientific community, including a common one known as MESA. We run these 1D models for the evolution of the star until we reach a point in that star’s life that we want a more detailed look at. We then take parameters like density, luminosity and temperature found by the 1D model and input them in our 3D model.
We use this approach because 1D models break down at a certain point. They become too simplistic to describe what’s going on in the star. 3D models can help us look deeper. However, they are very computationally demanding, so we can’t run them for the whole life of the star.
Our 3D model is based on code developed by Jim Stone and his collaborators. To this code, I added radiation transport algorithms that describe how photons move around. This movement is coupled to the existing magnetohydrodynamical algorithms to describe the magnetic processes at play.
Only in recent years have scientists really started including radiation equations in simulations, and usually this is done with a lot of approximations and assumptions because they are very complicated. We’re taking this one step farther by trying to solve the equations without using any assumptions.
The computational resources provided by the CCA make it possible for me to do my work, which requires a lot of computational time. At most institutions, you have to apply to get time on national supercomputers, which isn’t guaranteed. Here at the CCA, we have efficient and powerful supercomputing clusters in-house. Most of my work can be done just using the clusters provided by the center. The CCA also gives me the flexibility to work with students from other institutions, which makes for fun collaborations.

How long do your simulations take?
They typically take a few months to finish, but we do a lot of projects in parallel because we can’t just wait around for a single simulation to finish. We’re constantly going back and forth between projects and checking in on our running simulations to make sure they’re working properly and outputting the right information.
Why is it important to understand these systems?
Black holes play a very important role in all kinds of cosmic phenomena we see in astronomy.
Light emitted by an accreting black hole at the center of a galaxy can be more luminous than light emitted by the galaxy’s stars. The emission coming from black holes also plays an important role in regulating the growth of the galaxies across the universe.
Many of the high-energy objects we see in the nearby universe include either black holes or neutron stars. Such compact objects are very useful for understanding physics in strong gravitational regimes. By studying these objects, we get a look at some of the most bizarre systems in the universe.